By Mariana Meneses
The quantum computing revolution is on the horizon.
As scientists probe the mysteries of the quantum realm and push the boundaries of what’s possible, they propel us closer to a future of unimaginable computational power. In this article, we delve into the latest developments in quantum computing and explore how they are paving the way for a technological leap that promises to reshape industries across the globe.
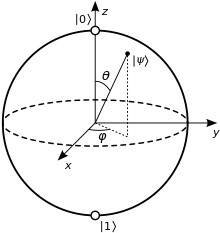
The qubit is a sphere in which signals distribute from three axes labelled x, y, and z, at an angle denoted by φ, to a probability amplitude of Ψ.
IBM has recently announced a 10-year plan to build a groundbreaking 100,000-qubit quantum computer in partnership with the University of Tokyo and the University of Chicago, investing US$100 million in a partnership to usher in a new era of quantum computing. While IBM currently holds the record for the largest quantum computing system with a 433-qubit processor, the forthcoming 100,000-qubit machine represents a paradigm shift in quantum capabilities.
Unlike today’s common computers which transmit data in bits that are in either a 0 (“off”) or 1 (“on”) state, but not both, quantum computers operate using quantum bits, or qubits.
Qubits operate in both 0 (“off”) and 1 (“on”) states simultaneously, a phenomenon known as superposition. This allows quantum computers to perform multiple computations at the same time encompassing all probabilities between both states, leading to exponential speedups for certain types of problems.
However, qubits are susceptible to decoherence, which is the loss of their superposition of 0 and 1 states that occurs when they interact with their surrounding environment and leads to errors in computations. To overcome this challenge and protect them from external interference, quantum processors are typically operated at cryogenic temperatures which reduce the errors of decoherence but are extremely expensive to maintain.
Rather than replacing traditional supercomputers, IBM’s ambitious endeavor seeks to merge quantum power with “classical” computing technology now in widespread use, in what they are calling “quantum-centric supercomputing.” By leveraging the strengths of both technologies, this fusion aims to address complex challenges that have remained unsolvable until now, achieving breakthroughs in drug discovery, fertilizer production, battery performance, and more.
Quantum computers, which harness the principles of quantum mechanics to perform calculations exponentially faster than classical computers, have the potential to revolutionize processes of encryption, optimization, and simulation.
However, the development of practical quantum computers has been hindered by the need for extremely low temperatures to maintain the delicate quantum states required for computation.
Room-temperature superconductivity, a rare phenomenon where certain materials can conduct electricity with zero resistance without supercooling, has been a long sought-after goal in physics. A superconductivity breakthrough could have significant implications, particularly in the realm of quantum computing.
Adapting nature’s technology to stabilize quantum computers
To theoretical physicist Michio Kaku, humanity is transitioning to a new quantum computing era and away from the binary on-off code operating in the bits of today’s classical computers. He believes that we are in the second stage of computer evolution, characterized by electricity-powered transistors and the development of the microchip, which helped shape today’s digital landscape. But today’s microchips use binary signals composed of either 0’s or 1’s, and this is not “natural.”
Instead, nature’s computations rely on electron waves, where electrical signals can exist both at single points as well as collections of probabilities in waves, and that’s what is expected in stage three of computing in the quantum realm.
Many new developments hint that we may be getting closer to this revolution.
For instance, recently Cornell scientists found a new state of matter which may enhance stability in quantum computers.
The new state of matter was found in a material called Uranium Ditelluride (UTe2) using a special microscope, and is called a “spin-triplet electron-pair crystal”. This crystal state could potentially change the way quantum computers work, making them more stable by helping to create topological superconductors. Topological superconductors are a special type of material that can conduct electricity without any resistance, and are the target of intense research by physicists to form the materials for ultra-stable quantum computers.
Recently, Stanford scientists, in collaboration with the University College Dublin, have developed a groundbreaking analog quantum computer capable of solving extremely challenging physics problems beyond the reach of even the most powerful binary supercomputers, offering potential insights into fundamental questions in physics and materials science.
Also called ‘Quantum Simulators,’ analog quantum computers are based on the technology and programmability of today’s binary computers, but use some quantum components to mimic a fully quantum system and solve complex problems that classical computers would lack the power to do on their own.
The researchers have demonstrated that this specialized analog computer, using quantum components in its circuits, can tackle complex quantum physics problems that were previously unsolvable. When scaled up, these devices could potentially help materials scientists to accurately design complex materials like room-temperature superconductors, which are currently beyond the capabilities of today’s computers and would transform a range of various technologies.
The recent widely-reported experiment revealed how a special crystal can conduct electricity with zero resistance at high temperatures.
The process showed that electrons in the crystal can achieve superconductivity by pushing each other into a flow without any friction, which was predicted by the “BCS” theory first advanced in 1957 and awarded the Nobel Prize 15 years later. If replicable, scientists hope to use this understanding to create materials that can conduct electricity without resistance at even higher temperatures, which would also make electronics and power lines much more efficient.
Boosting qubits with trapped ions, controlling fluctuations, and sound technology
Other recent quantum computer enhancements include the Enchilada Trap, a novel microchip that can transport up to 200 qubits by holding electrically charged ions. An upgrade from its predecessor the Roadrunner Trap, the Enchilada Trap holds the potential of reducing the problem of decoherence and enabling more complex calculations by future quantum computers.
Plus, at MIT, scientists have discovered a method to manipulate quantum randomness, offering potential for scientific advancements by harnessing controlled vacuum fluctuations.
Vacuum fluctuations are tiny movements that happen even in empty space and that are apparently random and hard to predict. Controlling these fluctuations could help researchers in many fields, such as predicting earthquakes and exploring space through “probabilistic computing”, a form of calculation that can give a range of possible outcomes instead of just one answer.
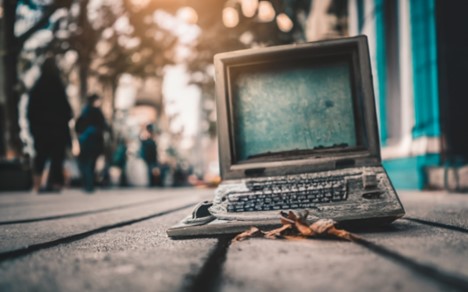
The quantum revolution is bound to redefine our technological landscape. Image generated using Ideogram AI.
And, in California, scientists at Caltech have created a new way to store information using sound, using a small machine that converts electricity into sound and back and offers a more efficient way to store quantum information and increase its longevity.
The method translates electrical quantum states into sound and vice versa by utilizing phonons, which are the sound equivalent of the photon, which is the particle of light. This avoids the energy loss associated with previous methods, enabling longer storage durations that could represent a significant advancement in the field of quantum computing.
Quantum computing is already generating breakthroughs in many fields.
For instance, in Australia, scientists at the University of Sydney used a quantum computer to study chemical actions that are vital in many natural processes like photosynthesis and vision which occur so quickly that it’s difficult to determine the sequencing of cause and effect.
The quantum computers allowed scientists to slow down these processes by a factor of 100 billion times to see what was happening, broadening our understanding of how important chemical reactions work.
These are just a few selections of recent breakthroughs in a fast-evolving field.
As quantum computing continues to push the boundaries of what’s possible, the potential applications are potentially boundless. From revolutionizing cryptography and drug discovery to optimizing supply chains and tackling complex simulations, the quantum computing revolution holds the promise to reshape our world in ways we can only begin to imagine.