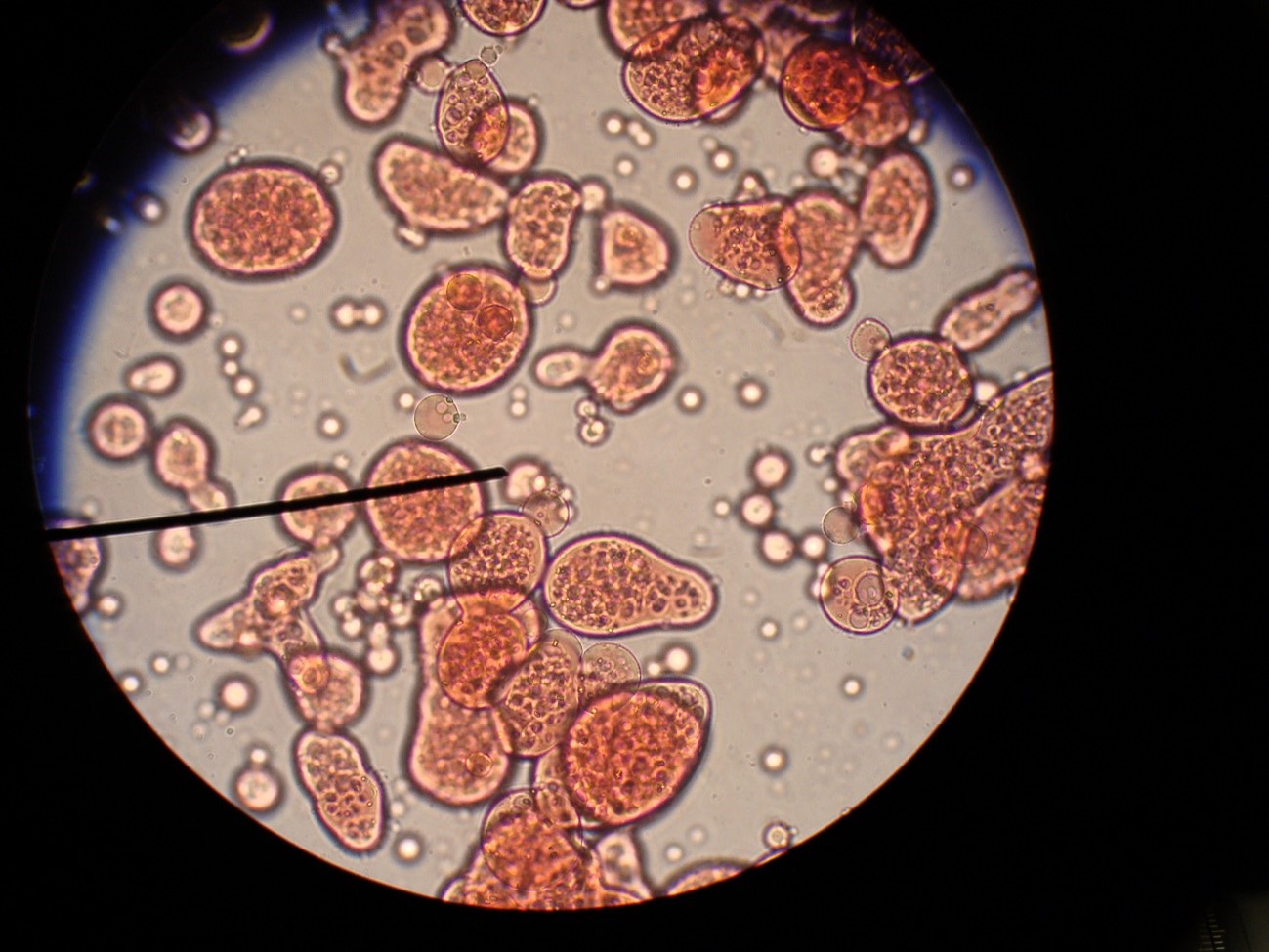
According to the Oparin-Haldane theory, organic molecules formed self-replicating agglomerates at the beginning of life on Earth.
By Saulo Silvestre
Our best understanding of how life on Earth originated is based on the idea that, approximately 3.5 billion years ago, certain conditions on our planet caused the spontaneous formation of organic molecules such as lipids, sugars, amino acids, and nucleic acids.
This idea was proposed independently by two scientists in the 1920s, the British geneticist John Haldane and the Russian biochemist Aleksandr Oparin. According to their theories, these organic molecules formed self-replicating clusters or agglomerates, which eventually evolved into the first living cells.
However, the path from agglomerates of organic molecules to the highly complex life forms spanning Earth today is full of significant evolutionary leaps, some of which scientists all over the world are still striving to understand. One of the earliest and arguably most significant of those leaps is the appearance of multicellular organisms.
Over the past hundred years, scientists have observed the spontaneous formation of organic agglomerates under experimental conditions designed to simulate the early Earth’s environment.
Notably, American chemists Harold C. Urey and Stanley Miller were the first to conduct these experiments in 1953. More recently, in 2013, Dr. Thomas M. McCollom recreated the experiment using conditions that more accurately reflected the early Earth, and managed to produce various organic molecules, including amino acids and nucleotides. In essence, the experiment demonstrated – and the scientists could observe – the emergence of all the fundamental building blocks of living cells.
But why would evolution favor the development of multicellular organisms in the first place?
Even though our human perspective may give the impression that multicellular organisms are the prevalent life form on Earth, in reality most life on Earth is unicellular, including bacteria, protozoans, many algae, and fungi species. Unicellularity is not only the oldest but also the predominant type of organism on the planet.
The increased complexity of multicellularity has some evolutionary drawbacks that leave us wondering, “why was it worth the effort?”
For example, when cells combine and give up their unicellular way of life, in many cases they may become “somatic,” which means they will no longer pass on their genes through reproduction. Additionally, the agglomeration of cells may increase competition for resources, and by combining to form a single organism, the cells tie their fate to one another. These drawbacks represent potential significant costs to the fitness of the individual cells involved.
However, as is often the case in nature, whether the outcome of something will be beneficial or not heavily depends on the context. Dr. Roberta May Fisher, a researcher at the University of Copenhagen, observed that unicellular algae could form multicellular clusters to defend against high densities of microscopic predators. Additionally, under certain conditions, multicellular organisms may move and forage better, which might tilt the balance between the costs and benefits of this evolutionary strategy.
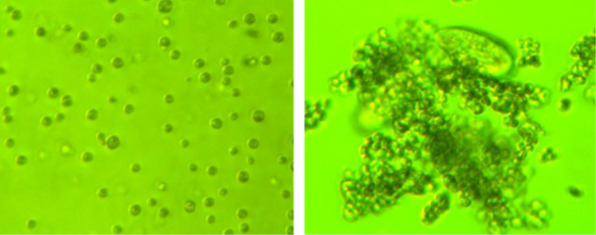
Examples of the algae studied by Dr. Fisher. On the left, algae unicells when predators are absent, and on the right, the formation of a multicellular cluster in the presence of predators. Image: Fisher et al. (2016).
In a 2021 study, a team of researchers led by Dr. William Ratcliff at the Georgia Institute of Technology reported that an originally unicellular lineage of yeast evolved into multicellular clusters that were visible to the naked eye in less than two years under experimental conditions.
Unlike Dr. Fisher’s observations, which demonstrated the ability of the algae to adopt different life strategies to accommodate local pressures, the experiment with yeast documented the evolution of a lineage from unicellularity to multicellularity. This breakthrough in the study of the mechanisms behind the origin of multicellularity resulted from almost a decade of research by the team.
During his doctoral research, Dr. Ratcliff and colleagues at the University of Minnesota conducted an experiment to promote the evolution of unicellular yeast into multicellular organisms.
During 60 consecutive days, they repeatedly shook tubes with yeast growing in culture and used the first yeast settling on the bottom to start new cultures. By doing this, they favored the reproduction of the yeast that would sink faster and, in a matter of weeks, lineages of yeast that remained attached after multiplying – forming clumps that settled quicker – emerged. Dr. Ratcliff named these clusters “snowflake yeast” because of their shapes.
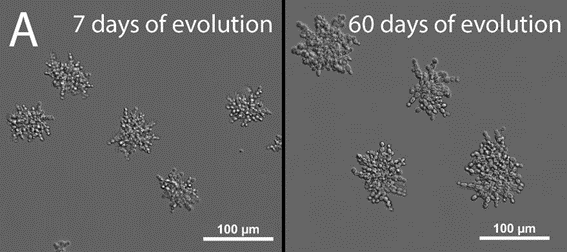
Snowflake yeast. Image: Pentz et al. (2015).
But even though the snowflake yeast rapidly achieved multicellularity, the team were unable to grow it beyond the microscopic level for nearly a decade.
None could find the key to the next step in the evolutionary journey of snowflake yeast until Dr. Ozan Bozdağ, at the time a postdoctoral researcher in Dr. Ratcliff’s laboratory, came up with a solution.
Cells use oxygen to break down sugar for massive energy payouts. The alternative for that would be fermentation, a much slower and less energy-efficient process. Dr. Bozdağ suggested that oxygen could be limiting the size of the clusters. Oxygen diffuses through cells at a fixed rate, so as the clusters grow, the cells in the interior receive oxygen at a much slower pace than the exterior, if at all. So, the team at Dr. Ratcliff’s laboratory recreated his original experiment, including a group of snowflake yeast that, thanks to a specific mutation, could not use oxygen and relied completely on fermentation. This difference took access to oxygen out of the equation for the survival of the yeast.
For 600 consecutive days, Dr. Bozdağ selected the first yeast that settled to the bottom of the test tubes after stirring. The clusters doubled in size in the first 100 days, and after around 350 days the first cluster visible to the naked eye emerged in one of the tubes with the mutant yeast. Around the 400th day, all five tubes with mutant yeast had clusters visible to the naked eye, as big as 20,000 times their initial size.
The researchers also noticed that with the increase in size, signs of differentiation also emerged. The yeast cells evolved to more elongated shapes, and the branches of the clusters tangled around each other, making them at least 10,000 times more resistant to breakage.
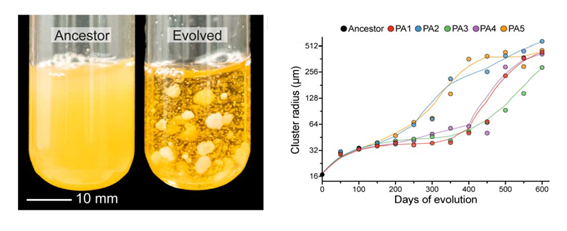
Evolution of multicellularity in five snowflake yeast populations. Image: Bozdağ et al. (2021).
Cell differentiation is a game-changer in the evolution of multicellular organisms.
Differentiation is the process in which the cells constituting the organism develop different specializations, forming tissues with complementary roles in the organism.
By focusing on specific functions, like muscle cells that facilitate movement, they often lose other abilities, like obtaining and digesting food and become dependent on the other cells of the organism to survive. The changes observed in the yeast cells by Dr. Bozdağ suggest that the evolutionary potential of the snowflake yeast in this experiment surpasses the simple growth of the clusters. Now, the team and the scientific community as a whole are looking forward to seeing what new changes may emerge in the snowflake yeast as the experiment continues.
Paleontological and geological evidence suggests that life on Earth emerged almost as soon as the minimum conditions for life were present. The transition of the snowflake yeast from unicellularity to multicellularity indicates how early life forms might have made this evolutionary leap in the past. It also explains to some extent how such a leap could have independently occurred many times in Earth’s history.
As demonstrated by Dr. Ratcliff’s team, once proper conditions meet specific selective pressures, evolution is bound to happen. Evolution, much like life itself, is a matter of opportunity.
Original article: Single Cells Evolve Large Multicellular Forms in Just Two Years