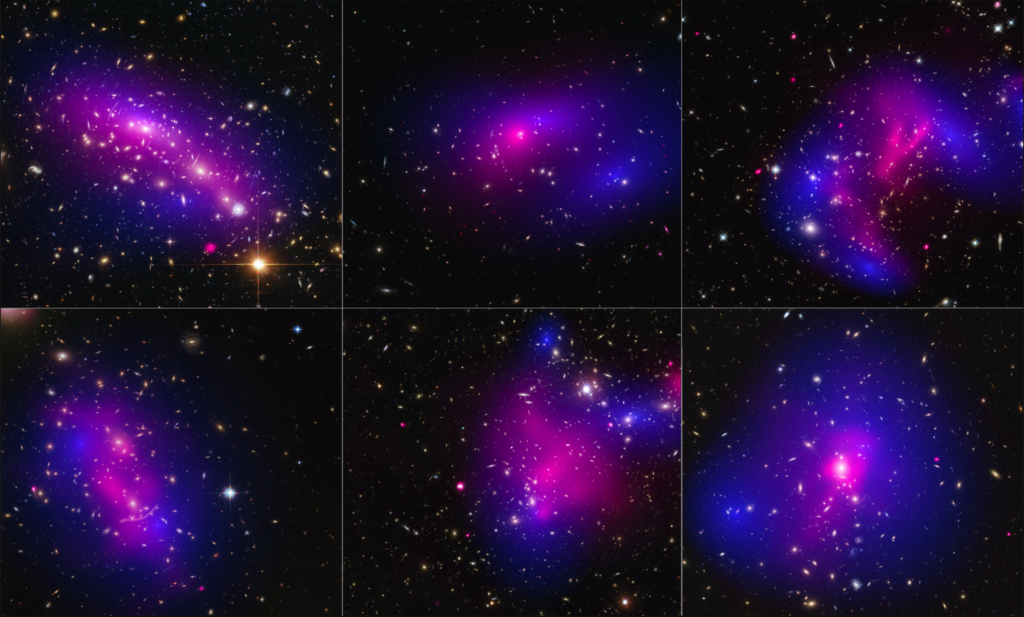
NASA has been searching for clues to identify Dark Matter. The image shows six different galaxy clusters from NASA’s Hubble Telescope (blue) and the Chandra X-ray Observatory (pink), in a study of how dark matter behaves in colliding clusters of galaxies. Credit: NASA and ESA
By Mariana Meneses
Dark matter remains a mysterious and theoretical type of matter that scientists believe makes up around 85% of the total mass in the universe.
Dark matter is referred to as “dark” because it doesn’t seem to interact with the electromagnetic field, which means it doesn’t absorb, emit, or reflect electromagnetic radiation. Because it’s “dark”, as it does not directly interact with light, it’s incredibly challenging to detect.
Several astrophysical observations strongly suggest the presence of dark matter, including gravitational effects that cannot be explained by our current understanding of gravity unless there is more matter than what we can see. This leads most experts to believe that dark matter is widespread and has played a substantial role in shaping the structure and evolution of the universe.
The first evidence supporting the existence of dark matter came from calculations that showed that galaxies would behave very differently if they didn’t contain significant amounts of invisible mass. Further evidence was added later, such as observations related to gravitational lensing – which refers to the way light bends around massive objects – and the cosmic microwave background, which is the impenetrable radiation of the early universe that marks the limit of how far back in time our telescopes can see.
Other evidence of dark matter has come from astronomical observations of the structure of the observable universe, the formation and development of galaxies, the distribution of mass during galactic collisions, and the movement of galaxies within galaxy clusters.
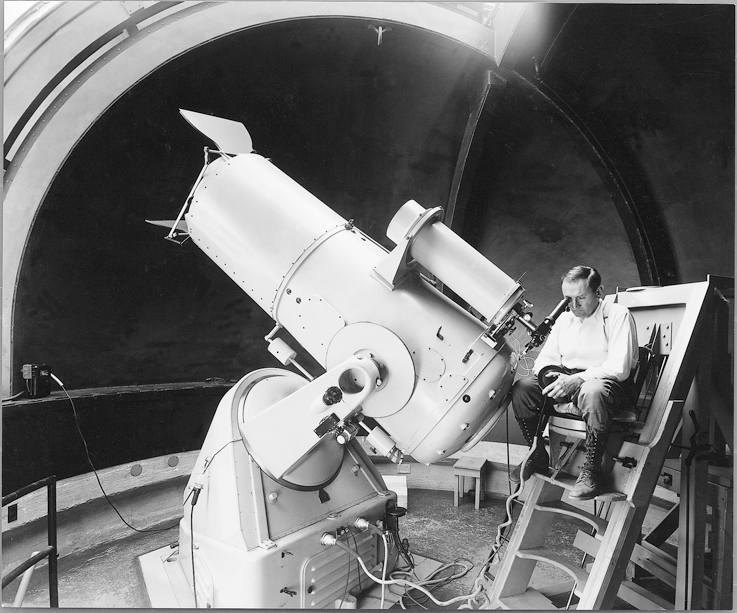
“Dark matter’s existence was first inferred by Swiss American astronomer Fritz Zwicky, who in 1933 discovered that the mass of all the stars in the Coma cluster of galaxies provided only about 1 percent of the mass needed to keep the galaxies from escaping the cluster’s gravitational pull.” (Britannica). Image: Zwicky Meeting
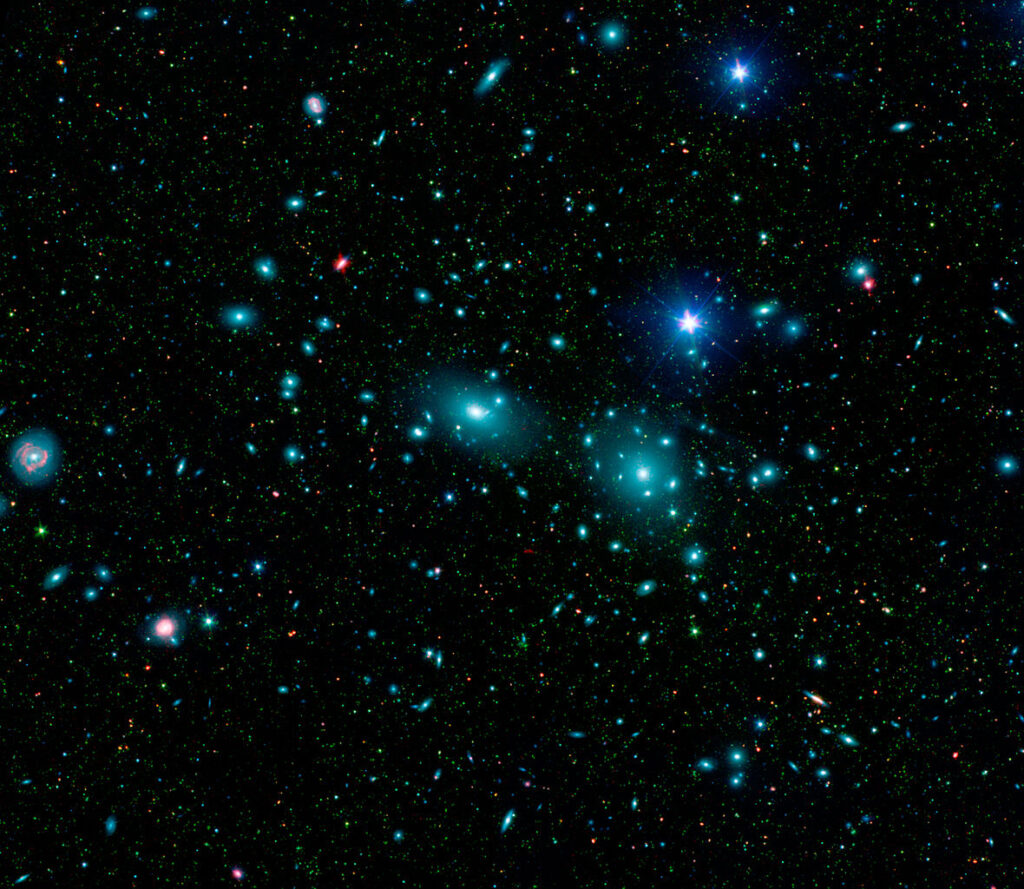
“This false-color mosaic of the central region of the Coma cluster combines infrared and visible-light images to reveal thousands of faint objects (green). Follow-up observations showed that many of these objects, which appear here as faint green smudges, are dwarf galaxies belonging to the cluster. Two large elliptical galaxies, NGC 4889 and NGC 4874, dominate the cluster’s center.” Image: NASA / JPL-Caltech / L. Jenkins (GSFC)
In the standard model of cosmology, the total mass and energy of the universe is divided into 5% ordinary matter, 26.8% dark matter, and 68.2% in an invisible form of energy called dark energy.
This means that dark energy and dark matter together account for a whopping 95% of the total mass and energy content of the observable universe. The scientific search for the nature of dark energy is ongoing at the same time as researchers are probing the structure, cause, and behavior of dark matter.
Directly observing dark matter remains an ongoing challenge mainly because it rarely interacts with ordinary matter and radiation, except through gravity. Some argue that dark matter may be composed of subatomic particles that have yet to be discovered, like weakly interacting massive particles (WIMPs) or axions. Numerous experiments aimed at detecting and studying dark matter particles directly are underway, but as of now, none have succeeded.
In November 2023, a team of researchers led by Dr. Ethan Nadler, from the University of Southern California, presented a new theory on an idea called “self-interacting dark matter” (SIDM) that suggests dark matter particles interact with each other through a mysterious force.
The team found that SIDM can explain two puzzles in space.
One puzzle is about dense dark matter in big galaxies, as observed from the strong gravitational effects of dark matter clusters. The other is about low-density dark matter in faint galaxies. To support this idea, they used computer simulations to show how self-interactions would affect the density of dark matter in different parts of galaxies. They believe SIDM might be a better explanation than the one we’ve had until now.
The term “faint galaxy” encompasses two distinct concepts based on context. On one hand, it refers to Faint Blue Galaxies, characterized by their small size, inconspicuous appearance, and a prevalence of scattered blue stars with fewer red stars. On the other hand, in the context of the early universe, a faint galaxy denotes a distant galaxy that is challenging to observe due to its faintness. Whether describing galaxies with lower matter density or those distant and challenging to detect, the term “faint” pertains not to physical distance but to the apparent brightness when observed from Earth.
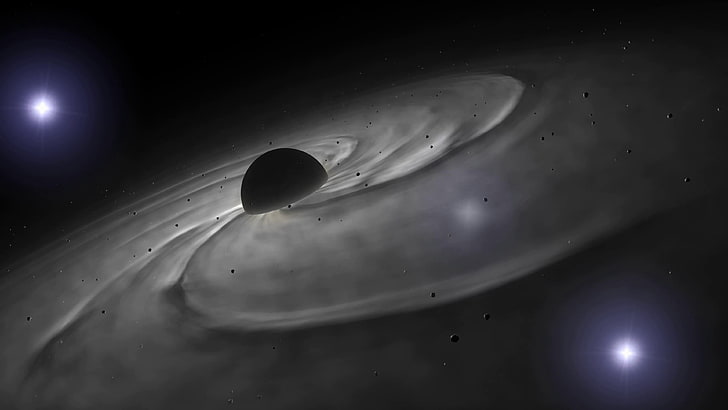
Artist’s rendition of a Dark Star. Image: Wallpaper Flare
Dark stars are a unique type of celestial object that might have existed in the early universe before regular stars could form.
These cosmic phenomena, fuelled by dark matter, could potentially be responsible for the existence of our universe as we know it today.
Unlike what happens in “regular” stars, like our Sun, dark stars would not be powered by nuclear fusion, but instead by the self-annihilation of dark matter.
This means that, unlike the shining stars we see in the night sky, dark stars would contain a high concentration of dark matter, producing heat through a process where dark matter particles annihilate each other. This heat would have a crucial role in preventing these dark stars from collapsing into the compact and dense structures we see in contemporary stars. This, in turn, would prevent the initiation of nuclear fusion reactions among the atoms of ordinary matter within them.
“Annihilate each other” denotes a unique process where dark matter particles interact, transforming their mass into energy. This distinguishes it from conventional particle collisions, which usually involve alterations in direction or state rather than mass-to-energy conversion. Referred to as “self-annihilation,” this process involves dark matter particles interacting among themselves rather than with other particle types. The energy generated through this annihilation is believed to contribute to the heat preventing dark stars from collapsing.
Recently, the James Webb Space Telescope spotted some objects in the early universe that could be dark stars, which until now are one of a number of different ideas. But if they turn out to be real, they could help us learn more about dark matter and how stars were formed in the early universe.
According to Scientific American, the candidate stars seen by the JWST will have to provide more evidence before their nature can be confirmed.
In the universe’s past, which we are peering further into and discovering more of with the help of the JWST, dark stars could have glowed a billion times more luminously than the sun and grown to a million times its mass. Researchers report that at least three far-off objects observed by the JWST and previously identified as galaxies could each be single, supermassive dark stars. JWST’s technology is sufficient to do that job, but researchers need more observation time.
The potential discovery of dark stars holds the power to revolutionize our comprehension of the early universe on multiple fronts.
Should researchers indeed provide evidence supporting their existence, it would change our understanding of the universe’s formative times. Dark stars could quickly become top candidates for being the first stars to exist, having taken shape approximately 200 million years following the Big Bang.
Understanding the mysterious beginnings of our universe and how the first stars formed is crucial for understanding the overall picture of the cosmos we see today and the complex events that occur within it.
Craving more information? Take a look at these recommended TQR articles:
NASA’s Hubble Space Telescope and Chandra X-ray Observatory
Does the Universe Have a Holographic Memory?
James Webb Space Telescope Advances Search for Signatures of Life
Space Exploration Technology Opens A Window On Time
James Webb Space Telescope: Hubble’s Successor May Rewrite the Physics Books