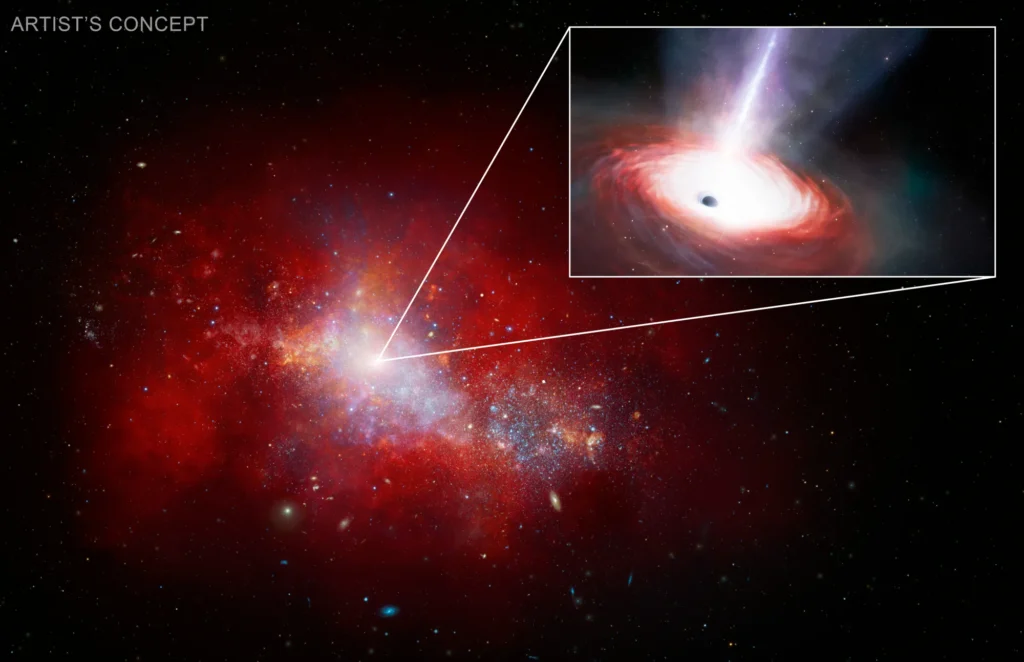
An illustration using data from NASA’s James Webb Space Telescope and the Chandra X-ray Observatory depicts a low-mass supermassive black hole named LID-568 at the centre of a dwarf galaxy whose light took 12.3 billion years to reach us. The black hole is drawing in matter at an incredible rate, 40 times the theoretical limit. Image from NASA by NOIRLab/NSF/AURA/J. da Silva/M. Zamani.
By James Myers
It was long thought that the physical universe came into being with the Big Bang 13.8 billion years ago, and that black holes didn’t form until billions of years later, after stars ran out of fuel and collapsed. That version of history is being seriously challenged, however, by data from the latest telescope technology that’s showing a universe at the dawn of time very different from what we thought it was.
The James Webb Space Telescope (JWST) was launched by NASA on December 25, 2021, and from its station about 1.5 million kilometres from Earth is collecting infrared light waves to provide incredibly precise images of the universe from its early days to the present. Combined data from the JWST and the Chandra X-ray Observatory, which detects x-rays generated by intense heat from strong magnetic fields, extreme gravity, or explosive forces, detected a black hole in a dwarf galaxy 1.5 billion years young. What scientists observed about the evolution of the universe came as a surprise.
Black holes are objects with massive gravity that are now known to exist beyond the limits of space and time in the middle of almost all galaxies, including our Milky Way.
The JWST and Chandra provided infrared and x-ray data from the young galaxy to reveal the incredible rate at which its central black hole, named LID-568, was attracting physical matter.
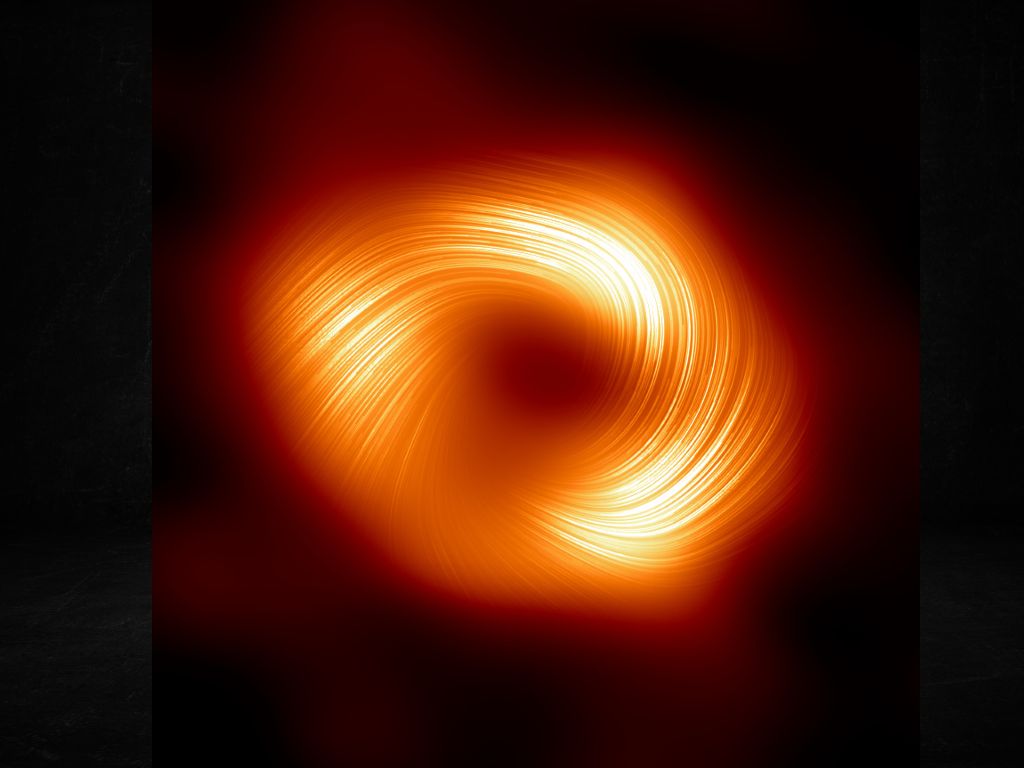
Image of the supermassive black hole called Sagittarius A* at the centre of the Milky Way Galaxy taken by the Event Horizon Telescope, with lines overlaid to show the orientation of its magnetic polarization. Released in March 2024, this image represents the first time that astronomers have been able to measure polarization, a signature of magnetic fields, at a distance close to the edge of Sagittarius A* and the black hole’s event horizon, where space and time end. Image: Event Horizon Telescope Collaboration
Matter was being drawn in by black hole LID-568 at a rate 40 times faster than the theorized Eddington limit, which is based on a black hole’s gravitational force and the amount of light that it can emit from objects that fall into it. Far exceeding the limit, black hole LID-568 experienced a very quick growth spurt in the young universe. This may explain one of the anomalies of early black hole formation, when stars were too young to have imploded with enough force to open a hole in the fabric of spacetime.
The oldest galaxy in the universe has now been observed, together with “little red dots.”
Using the JWST, a team of astronomers from the University of Arizona has set a record by observing the oldest known galaxy, innocuously named JADES GS-z14-0. The wavelengths of its light are stretched so long into the red spectrum – which is the oldest light in the universe – that in May 2024 the galaxy was dated to 300 million years after the Big Bang. At only 2% of the universe’s current age, it was a toddler in the continuing expansion of spacetime and is as far back in time as we have been able to detect.
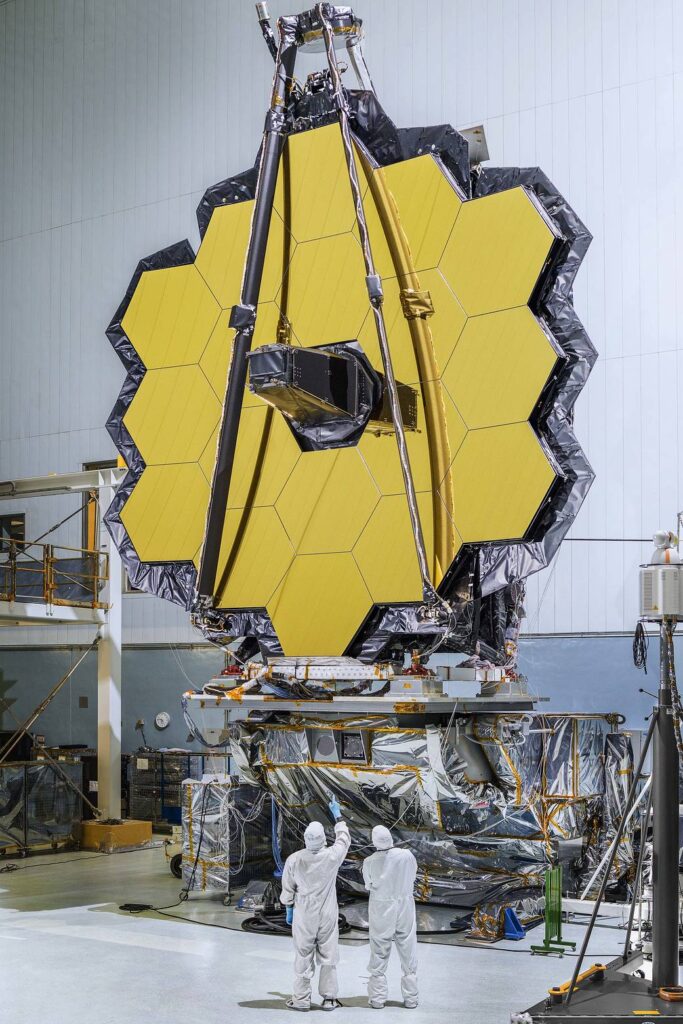
Photo of assembly of the mirrors for the James Webb Space Telescope taken in 2016. Image: NASA, on Wikipedia.
Dr. Kevin Hainline, who was part of the astronomical team that observed JADES GS-z14-0, stated that the galaxy is surprising not only for its age but for how “stupendously bright” it was, in spite of its vast distance in time and space from us. The galaxy is five times brighter than the second-oldest known galaxy.
Dr. Hainline told the University of Arizona News that the galaxy “was discovered in an area of the sky that appears to be as large as a grain of sand held at arm’s length, so galaxies like it could potentially be common.”
Among the most significant findings to date of the JWST are what are known as “little red dots.”
Little red dots are strangely bright galaxies with so many brilliant objects clustered in them that scientists wonder how such a high density could exist without collisions. If their light is from stars, scientists wonder how so many stars could have acquired such great mass in the early universe. Alternatively, if their light is generated by the friction of objects falling into black holes, scientists question why they can’t detect x-rays and radio waves which normally signify the presence of black holes.
Dr. Josephine Baggen of Yale University told New Scientist Magazine that the little red dots are “so compact, it was kind of a shock the first time we measured these sizes. They’re about 100 times smaller than a typical galaxy that we see today, like the Milky Way, but it’s possible that they have the same stellar mass. That’s a big issue.”
In a paper published this August, Dr. Baggen and co-authors noted, “It also remains to be seen whether these ultra compact galaxies are stable against gravitational collapse; it may be that parts of the galaxies collapse into supermassive black holes while the rest expands.”
There are three theories that receive widespread acceptance for how galaxies became so bright so quickly after the universe was born in the Big Bang. One is that early stars weren’t nearly as massive as they are now, but they made up for low mass with extreme brightness. The theory doesn’t work well, however, with the initial mass function, which is the standard correlation of a star’s mass and brightness that is used in computational models.
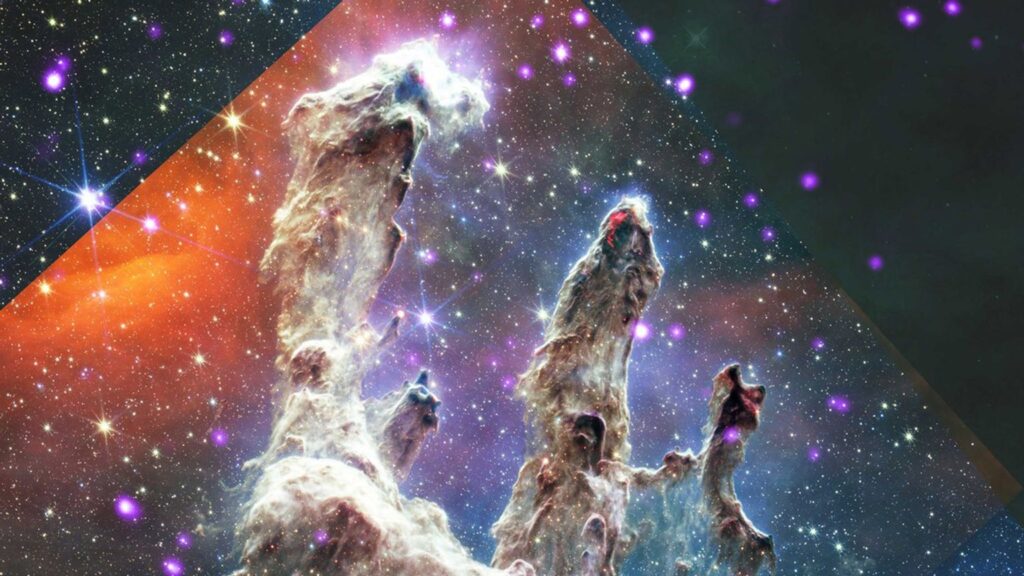
Image of the Eagle Nebula, taken by the Hubble Space Telescope. Nebulas are dense clouds of gas, sometimes called “stellar nurseries,” in which stars form. Image: NASA.
Another theory is that stars were forming so rapidly in the early universe that concentrations of their brilliance produced more early light than lower concentrations of stars now do. If young stars evolved quickly, they could have run out of fuel and imploded in ultra-bright supernova explosions, which would have added to the light of the young galaxies.
A third theory is that star formation in the early universe was more efficient than it now is. Stars form when cold clouds of hydrogen, which is the most abundant element in the universe, condense with such intense pressure that nuclear fusion occurs, joining hydrogen atoms to form helium which is the fuel that powers our sun and trillions of other stars in the universe. The theory suggests that hydrogen was more concentrated in the smaller area of the young universe, and therefore more easily and quickly condensed to form stellar cores.
Along with these theories of star formation, another theory of the birth of early black holes is emerging. Instead of forming only from the collapse of stars at the end of their lives, some believe that black holes in the early universe might have formed from the collapse of massive gas clouds. One difficulty with a theory like this, however, is obtaining an accurate measurement of a black hole’s mass. While some scientists gauge mass from the hole’s electromagnetic output, others argue that method of measurement is inaccurate or requires confirmation with other measures.
What will be learned as more light is shed on the early universe?
Computational modelling of the evolution of matter in the early universe has so far depended on correlating the mass and brightness of stars, but discrepancies observed by the JWST and Chandra telescopes might require development of a different approach. Key to establishing the correct method for measuring will be a better understanding of the causes of the early universe’s expansion.
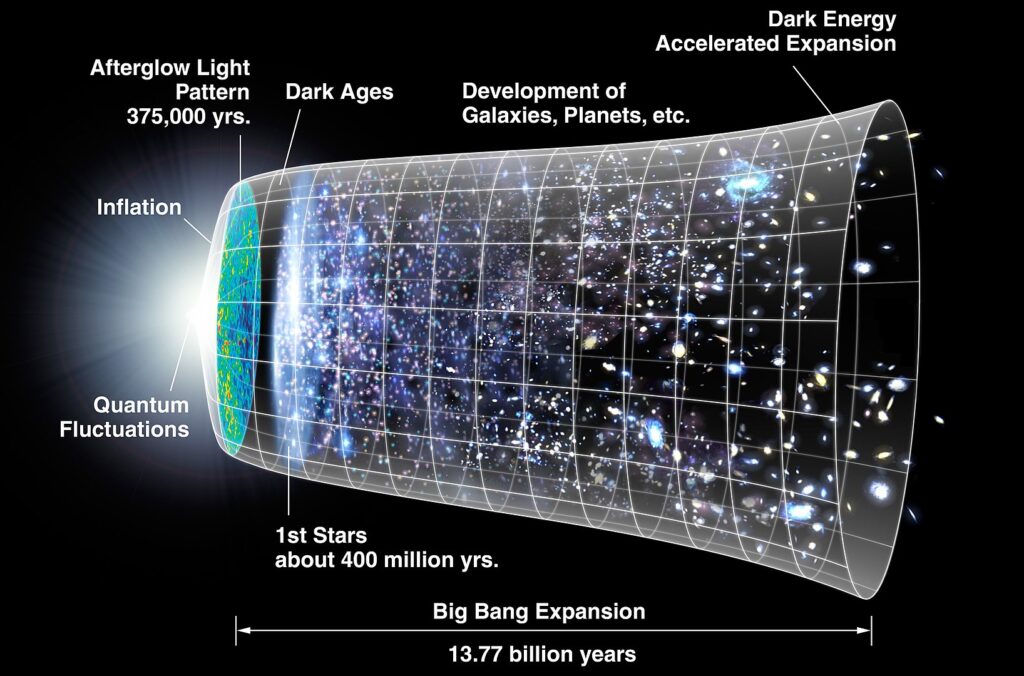
A representation of the evolution of the universe over 13.77 billion years. The band at the left marked “Dark Ages” depicts the earliest moment we can now probe, after a period of “inflation” produced a burst of exponential growth in the universe. (Size is depicted by the vertical extent of the grid in this graphic.) For the next several billion years, the expansion of the universe gradually slowed down as the matter in the universe pulled on itself via gravity. More recently, the expansion has begun to speed up again as the repulsive effects of dark energy have come to dominate the expansion of the universe. Illustration from NASA, on Wikipedia .
The rate of expansion of the universe, and the fate of a continually expanding universe, are ongoing questions that further observations of the evolution of the early universe could help to settle. Some theories, for example, hold that the universe will eventually expand into a “big freeze,” in which objects like suns and planets are so far away from each other that thermodynamic energy will no longer exist; the big freeze is sometimes referred to as the “heat death of the universe.”
In 1929, astronomer Edwin Hubble published his observations that the universe is expanding, which he detected by measuring the red-shifted light frequencies of galaxies against the constant light pulsations of Cepheid variable stars. Since then, the rate of expansion has been debated. What has come to be known as Hubble’s constant places the expansion at approximately 70 kilometres per second, which is about 3,000 times faster than a vehicle typically moves on a highway. New observations in 1998, however, indicate that not only is the universe expanding, but the rate of expansion is accelerating.
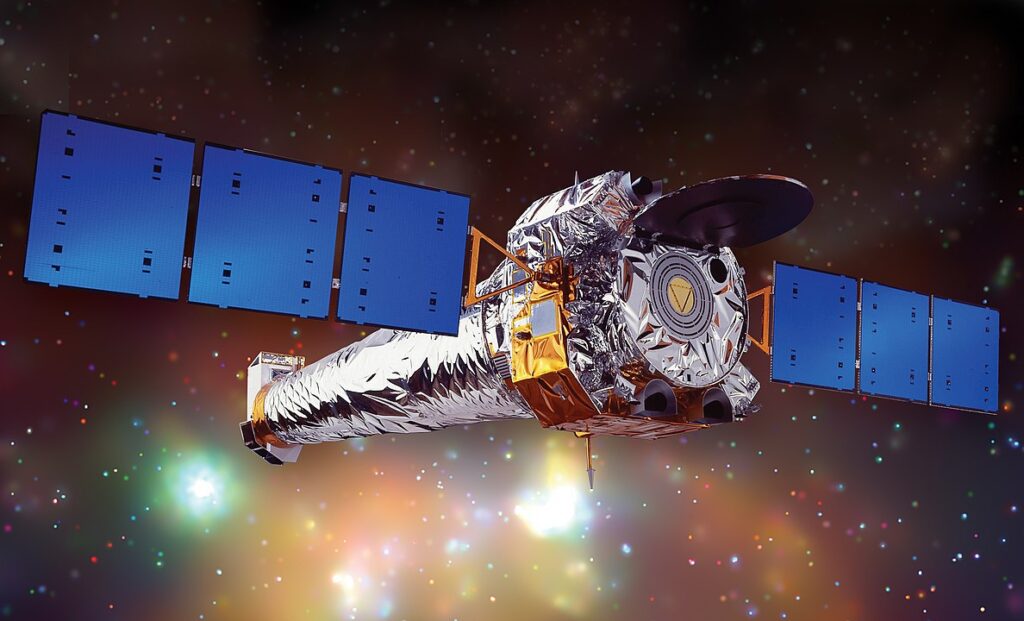
Artist rendering of the Chandra X-ray Observatory, data from which combined with infrared observations from the JWST to detect black hole LID-568. Image: NASA, on Wikipedia .
A more accurate measurement of expansion and acceleration could lead to further discoveries of the fundamental properties of energy and thermodynamics, potentially at the tiniest scale of the quantum. (For more on quantum thermodynamics, refer to The Quantum Feedback Loop podcast’s discussion with Twesh Upadhyaya).
Further observations of the evolution of galaxies, suns, black holes, and other objects in the universe might also yield a better understanding of the still-mysterious nature and origin of dark energy and dark matter. Together, dark energy and dark matter account for 95% of the mass and energy of the universe, but they are called dark because they’re not observable using existing equipment and can be detected only by their gravitational effect on physical objects. Dark matter is gravitationally attractive, causing objects to come together, while dark energy drives objects apart. Discovering how these forces contributed to shaping the early universe may resolve some major questions and open new avenues for science to exploit their properties.
While The James Webb Space Telescope and Chandra X-ray Observatory are raising questions about the evolution of the universe that we thought had been settled, they’re also pointing to some surprising answers. Other powerful observational technologies are coming online, like the Vera C. Rubin Observatory which is expected to begin operation in 2025, and the European Union’s Euclid telescope launched in 2023 with a mission of detecting the red-shift of light from galaxies. These may provide more answers than questions.
Whether it’s with questions or answers, we will come closer to understanding how the universe came to be and where it’s heading in time.
Your feedback helps us shape The Quantum Record just for you. Share your thoughts in our quick, 2-minute survey!
☞ Click here to complete our 2-minute survey