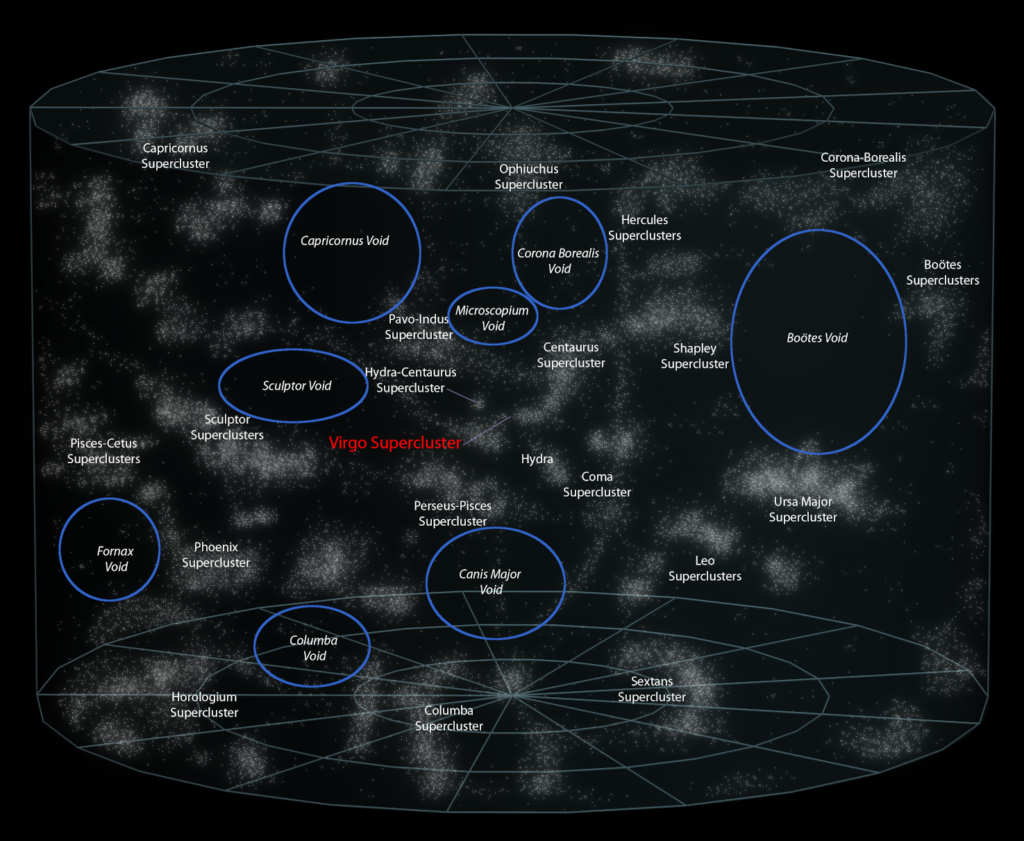
A map of galaxy voids in a cylindrical cross-section of the universe. The voids are ringed in blue. Image: Azcolvin429 on Wikipedia
By James Myers
Astronomers looking back in time to the light of the early universe are learning from the physical matter they detect, but they’re also uncovering the story of the young universe from cosmic voids of nothingness in space.
Galaxies, each of which is centered on a black hole, are areas of physical matter interacting by the force of gravity. Telescopes have shown that galaxies, of which there are at least 200 billion existing, connect throughout the universe in filaments. The filaments form a web-like structure in space, and there’s much to be learned from the spaces between these galactic filaments.
Voids among physical matter are yielding more information on several mysteries of physics. Voids are providing more insights into dark matter and dark energy, which our instruments can’t measure directly, but from their massive gravitational and expansionary effects on physical matter we believe comprise 95% of the mass and energy of the universe. Some scientists even say that voids might be the cause of dark energy.
Voids are also revealing more about neutrinos, those tiny unnoticed particles which pour out of the sun and shower through our bodies at the estimated rate of 100 trillion per second.
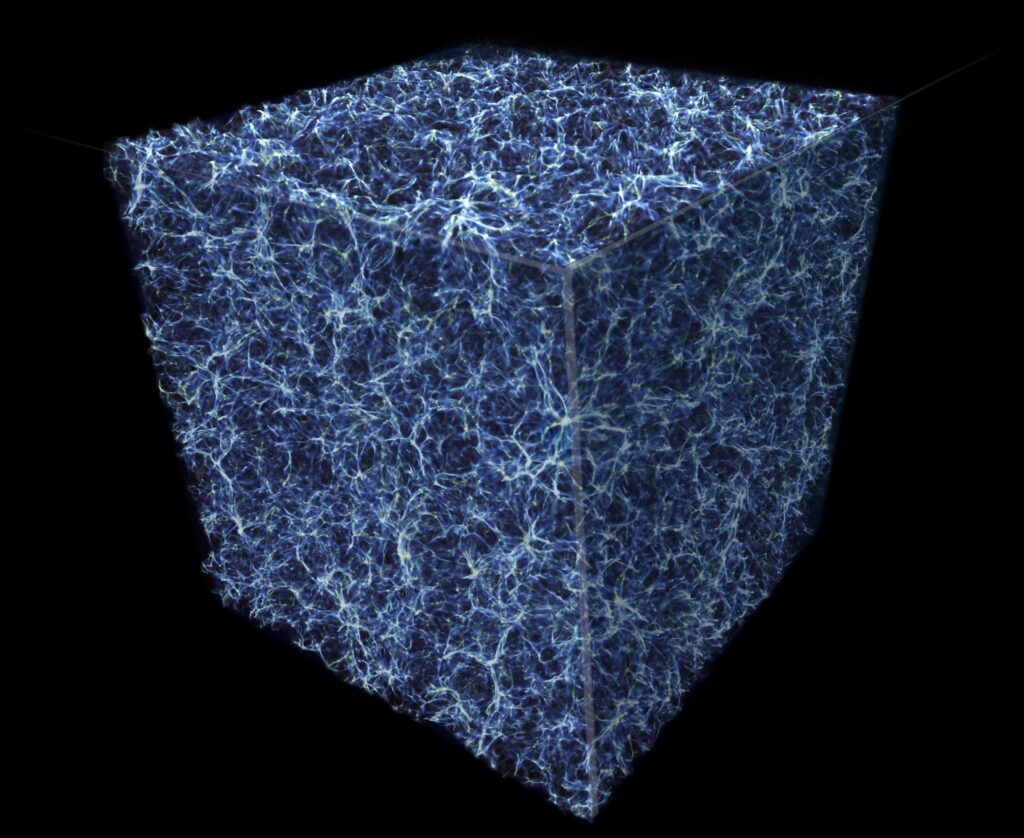
Simulation of a cubic section of the “cosmic web,” with the filaments representing mostly dark matter but also physical matter, containing voids between them. Image: NASA
Scientific American quotes Simons Foundation president and former Princeton University astrophysics chair David Spergel’s statement that, “Now is the right moment to use voids” for cosmology.
Also quoted is Benjamin Wandelt of the Lagrange Institute in Paris, who said, “Voids have really taken off. They’re becoming kind of a hot topic.”
The first void was detected in 1978, and since then technologies like the Sloan Digital Sky Survey that are mapping the universe have shown voids to be commonplace and extensive. It is estimated that voids occupy 90% of cosmic space.
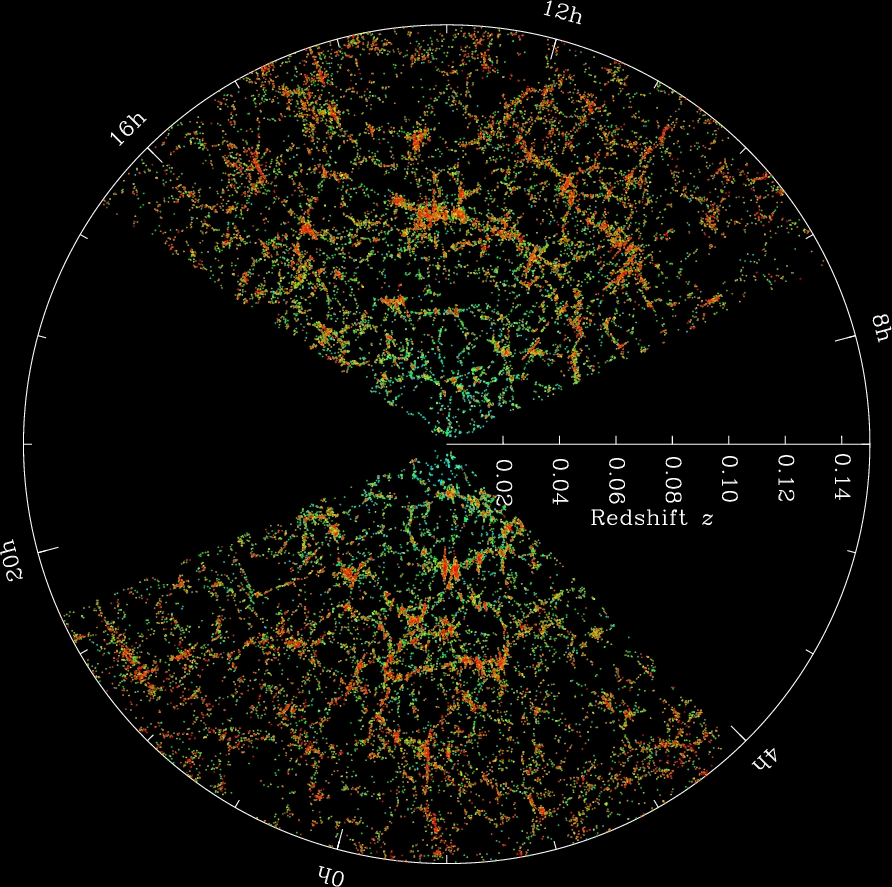
The Sloan Digital Sky Survey is mapping the universe. This image shows a cross-section of a map, with the Earth at the middle and a radius of 2 billion light years to the outer circle. Each point on the map is a galaxy. Image: M. Blanton and the Sloan Digital Sky Survey
The European Space Agency’s Euclid Mission, launched in the summer of 2023, is creating a highly-detailed map of the galactic filaments, and this year the Vera C. Rubin Observatory will begin a 10-year study of the cosmic structure.
The Euclid Mission includes around 100 scientists dedicated to studying voids, and as part of its mission will measure the sum of neutrino masses by comparing the statistical properties of void samples to theoretical predictions.
Since astronomer Edwin Hubble (1889-1953) first observed that the universe is expanding, we have been able to measure the rate of expansion by gauging the wavelengths of light at varying distances with the knowledge that as wavelengths stretch, or flatten, they become more red. Measuring the surrounding light reveals the velocity of the voids, which is the distance and direction in which the voids either expand or contract over a specified amount of time. Differences in velocities between the voids provides evidence of patterns in the pushing of dark energy and its relationship with the pulling of dark matter, and their interactions with physical mass that worlds like ours comprise.
The voids themselves are massive, with diameters typically between 10 and 100 megaparsecs which is a range from about 30,000,000 to 300,000,000 light-years across. For comparison, the Milky Way galaxy that we inhabit is a relatively minor 90,000 light-years across, and our nearest galactic neighbour, Andromeda, is about 2,500,000 light-years away.
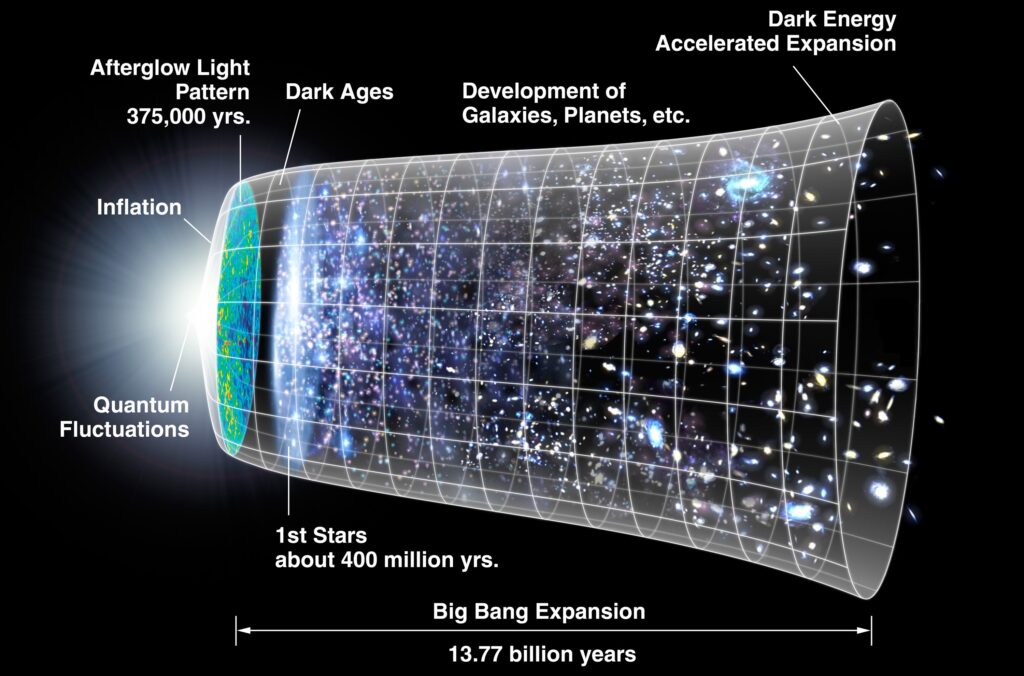
The timeline of the universe, beginning with the Big Bang, followed by the plasma of the cosmic microwave background in the first 375,000 years, after which a void existed before the formation of galactic filaments. Image: Wikipedia
The voids are thought to be the result of “baryonic acoustic vibrations” from the Big Bang.
We might imagine shockwaves pulsing out from the sudden, instantaneous explosion of all physical matter into the universe from a single, tiny point, and their vibrations forming rings that contain voids. As such, the perimeters of the voids would contain information of the initial state of the universe, and the Big Bang itself, which is thought to have occurred around 13.8 billion years ago.
In the same sense that we learn more about the universe by studying the behaviour of the matter swirling around a black hole, we can gain more clues by observing the borders of the voids.
The differences in the vibrations are thought to have been generated by quantum fluctuations from the Big Bang.
As a distinct ring of void forms with each quantum fluctuation, and each vibrational ring varies in size and position in relation to past and future rings, the rings of void could convey information about the sequencing of all vibrations that emerged from the Big Bang.
Uncertainty in Quantum Measurement
Can the voids tell us something about the quantum itself? The quantum is the smallest amount of energy in the universe that can cause physical change or be changed physically, but the quantum is notoriously difficult to measure. The void-causing quantum fluctuations are localized and apparently random differences in quantum energy, and are a product of Werner Heisenberg’s Uncertainty Principle in two respects.
The Uncertainty Principle says that the more information we have about an object’s position in space, the less information we have about the object’s momentum in time, and vice versa. We can’t precisely measure the position of a quantum in either space or time because every quantum is constantly in motion – and is therefore fluctuating. To be measurable, a thing has to be at a single point in space and time, but the quantum jumps continuously between both and never stands still. The Uncertainty Principle also says that there’s a minimum variability in our measurements of space and time, and it’s an important minimum because it has no limit. The minimum variability in any measurement is the Planck constant divided into a circle.
The Planck constant is the minimum measurement for the action of the universe’s energy. The Planck constant is named after physicist Max Planck, who called it the “quantum of action.” While Albert Einstein’s famous equation for energy E=mc2 is commonly known, energy can also be expressed in terms of the Planck constant, denoted by h, multiplied by frequency, denoted as f, as follows: E=hf. Although it’s a minimum, the Planck constant’s unlimited potential for measurement arises from its division into a circle, since circles have no beginning and no end and every point in a circle is equal to any other point in a circle. There’s no “there” and “elsewhere”, and no “past” or “future” in a circle, because any point in the circle has equal potential to any other point.
Since the measurement of a circle’s round circumference in relation to its straight diameter contains the famous value Pi, that mysterious ratio of 3.14159….. and on to infinite decimals (or at least beyond the trillions our computers have calculated), no exact measurement can ever be made. That’s because, mathematically and geometrically, Pi is both irrational (meaning that it never forms an exact fraction, or ratio) and it’s transcendental (meaning that it can’t be expressed in algebra).
Hence, the Uncertainty Principle prevails, when the minimum measurement of the Planck constant is distributed in a circle.
Dark energy and dark matter aren’t directly measurable using today’s technologies, although much is being learned about them from their effects on physical matter – which is measurable.
A team of scientists have theorized that the voids exist with a surface tension that applies pressure on surrounding physical matter and is the cause of dark energy. We might imagine such voids as expanding soap bubbles, with the stretching of their surfaces pressing on and causing motion in the surrounding water. The researchers suggest that the expansion of the voids causes a thinning of the physical matter they are in contact with, to the point where the voids merge and cause a change in the pressure they apply to the physical structures.
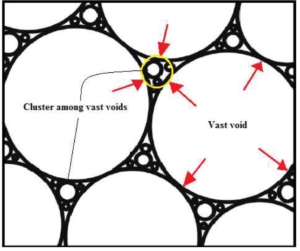
Schematic of voids (the black circles) applying force on physical matter (contained in the yellow circle), from “Surface Tension of Cosmic Voids as a Possible Source for Dark Energy” by E. Yusofi, M. Khanpour, M.A. Ramzanpour, and M. Mohsenzadeh.]
While voids contain some matter, they are devoid of large galactic structures and consist mostly of a vacuum of empty space.
The vacuum has an energy density called the cosmological constant, which is very small compared to all other natural energy scales, but remains a significant mystery.
As theoretical physicist Isabel Garcia Garcia explained to Steven Strogatz in Quanta Magazine’s Joy of Why podcast, while each quantum in the quantum field of a vacuum is itself a zero-point of energy, quanta fluctuate, or oscillate, in motion at varying frequencies, and it’s this fluctuation that gives energy to the vacuum. Vacuum energy is demonstrated in the Casimir effect, where two uncharged plates are placed in a vacuum whose energy pressure acts on the plates inversely proportional to the fourth power of the distance between the plates. The effect is subtle, and the plates must be extremely close because the pressure is so small, but the vacuum energy is nonetheless measurable and acknowledged.
Why is studying nothing physical, like the voids and vacuum, so important to modern physics?
As Isabel Garcia Garcia explains,
“In a sense, the vacuum is sort of like the starting point of any theory. It’s part of the definition of what your theory is. You define your vacuum state as the thing where nothing can be removed from it. In technical terms we sometimes say the vacuum cannot be annihilated, there’s nothing left to be removed, and on top of that you build everything else. So the properties of these vacuum states influence the properties of everything else that you’re going to build on top of it. They influence the properties of your theory at any other energy scale, so it’s sort of like the pillars of the rest of your theory that you’re going to use to describe nature.”
Does this mean that we get something from nothing?
Although no one has been able to make physical matter appear magically from thin air, knowledge of something can emerge from observing physical voids. What more will we discover from knowing nothingness?