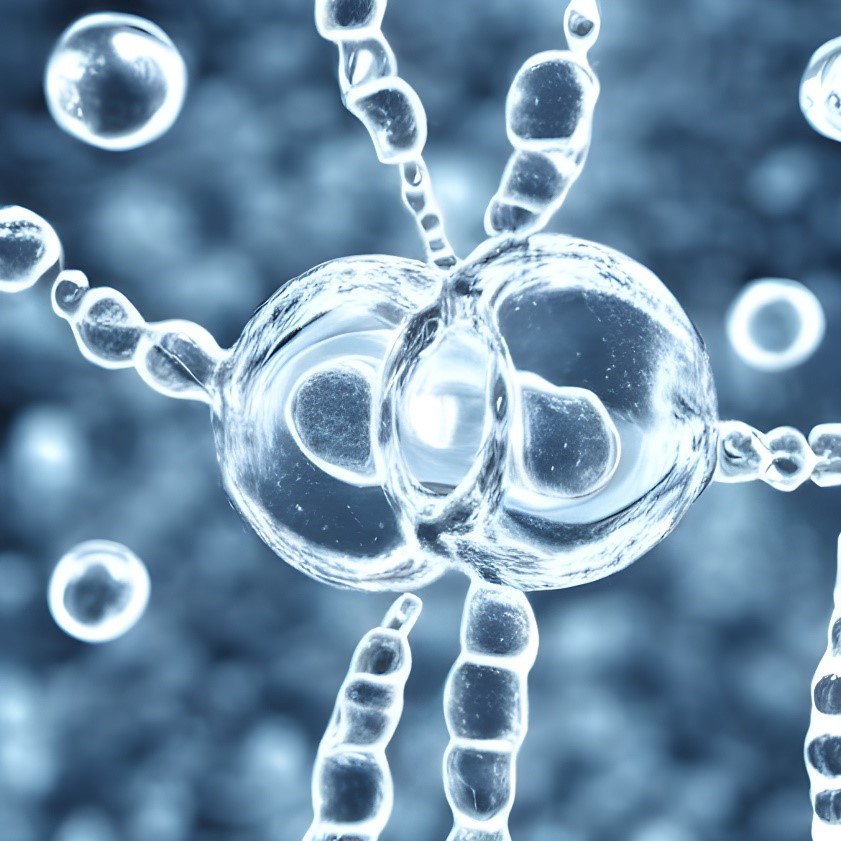
Image generated using DeepAI text-to-image generator.
By Saulo Silvestre
Cells are the smallest structural and functional units of life.
From unicellular organisms, like most bacteria, to organisms made up of trillions of cells, like humans, cells shape life as we know it. Countless reactions inside living cells create molecules, generate energy, promote growth and decay, and allow many other functions to occur continuously and simultaneously in our bodies at any time. These intracellular reactions are at the core of a cell’s purpose, and without them our bodies would simply not function.
Lacking anything close to consciousness, cells rely solely on molecules whose purpose is to provide chemical messages that tell them what to do and when to do it.
These signal molecules are the communication lines in this system, carrying instructions to receptors in the cells that set the cellular machinery into action. For a long time, we thought of this system as something analogous to electronic circuits, with information flowing in a tightly controlled manner beginning in the genes and ending with the traits expressed in the cells.
Although experiments with bacteria indicated that this analogy is sometimes accurate, it is not the case for more complex cells.
Over 20 years ago, Dr. Michael Elowitz, professor at the California Institute of Technology, was one of the first to design a functioning circuit in living cells. He and his mentor at the time, Dr. Stanislas Leibler, edited the genetic code of bacteria to induce controlled variations in their production of a fluorescent protein. This experiment produced bacteria that would visibly turn on and off, showing how the cell’s machinery can carry a command from the genes to the structures responsible for executing it, which is generally the way electronic circuits work.
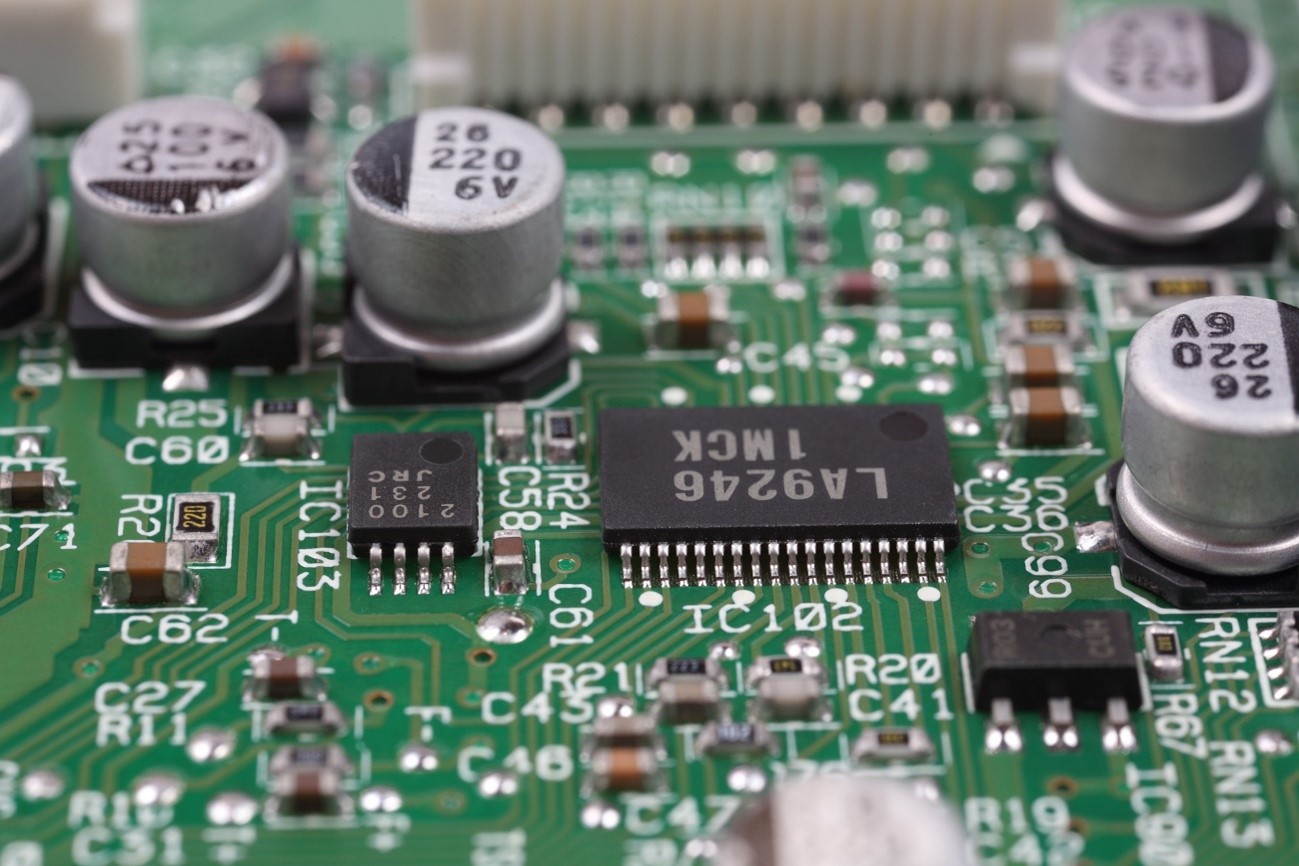
Electronic circuit. Photo: Pixabay
However, different results emerge when scientists attempt similar experiments using more complex, multicellular organisms.
By focusing on the protein interactions within a specific subset of cellular mechanisms in the development of the bodies of humans and other complex animals, the team led by Dr. Elowitz have discovered why. It turns out that traditional models vastly underestimated the true complexity of the networks of molecules that carry commands within and between cells.
Traditionally, researchers thought that signaling systems within cells worked as in a lock-and-key model, in which biological and chemical effects result from unique interactions between signal molecules and receptors.
Dr. Elowitz’s team’s observations revealed that instead of each outcome resulting from a specific signal molecule that uniquely binds to an associated receptor, signal molecules and receptors behave much more promiscuously. Each key fits multiple locks, and each lock matches with multiple keys.

Model of different system architectures. Image: Adapted from Su et al. (2021)
But what are the odds that, in such a system, we would consistently obtain the same results from a specific key or set of keys?
It doesn’t seem likely. However, somehow that is what happens inside living cells. What may seem like a chaotic system is the best way found by Evolution to convey the messages that are responsible for the fate of the cells and, ultimately, of the entire organism. The question is: why?
Dr. Elowitz’s team analyzed the growth factor proteins, which regulate how cells proliferate and differentiate into various tissues, like cartilage and the early brain. These signal molecules mediate the communication between cells but trigger different effects depending on the type of cells or stage of development.
From this clue, the researchers realized signal molecules are the messengers, not the message.
Each protein of the growth factor family has a slightly different structure. Likewise, the receptor proteins they bind to are built of varying combinations of subunits that fit together. Like Lego bricks, these components can be assembled in many ways, forming a system of combinations in which each signal molecule has different degrees of attraction to each receptor, defining how strongly they bind together and, consequently, the intensity of the outcome.
Some combinations may have nearly interchangeable effects, but just as likely they can have cumulative, opposite, or even independent effects. Also, the biological and chemical effects of different signal molecules are not fixed and vary according to cell types and the configuration of their receptors.
Christina Su, then Dr. Elowitz’s MD-PhD student, published an article showing how this complexity enables a small number of signal molecules to trigger a greater variety of effects in comparison to more specific architectures. Dr. James Linton, a researcher at Caltech, illustrates this logic using a parallel with our system of smell. We only have around 400 types of receptor proteins in our noses, yet they distinguish a seemingly incalculable variety of odors. That would be impossible if each receptor exclusively recognized one specific odor molecule.
Dr. Heidi Klumpe, another member of Elowitz’s group, compares this system to neural networks in which the components do not have fixed roles but, instead, the roles emerge from the connections established. The researchers even speculate that this might be the only feasible way for complex life to exist.
Most cellular components drift about inside the cell, making their encounters with molecules largely unpredictable.
If each element were wired specifically to another, it would seem as if circuit elements are randomly appearing in and disappearing from the network. Additionally, the way cells divide creates no guarantee that all the components will be present in the resulting cells.
A promiscuous system can more easily cope with mutations in its components, and, unlike the alternative, gaining a new function does not require the loss of another. The fluidity of the interactions in the molecular network decreases the susceptibility of cells to replication errors and facilitates the emergence of new capacities through evolution.
Knowledge of cellular communication may unlock the development of highly-effective drugs.
This new paradigm represents a significant shift in our understanding of the evolution of complex life forms and promises significant potential medical applications. Dr. Elowitz explained that “one of the challenges in ordinary medicine is that drugs can be very specific for a target protein, but that target protein may be nonspecific in terms of the cell types in which it is expressed.”
Currently, we have drugs that accurately target specific proteins, but our knowledge of whether and how these substances affect different tissues is notoriously limited. A more in-depth understanding of the mechanisms regulating the functions of our cells provides us with tools to assess the molecular interactions inside our bodies, including those involving pathogens and diseases, and to design even more effective medical treatments.
Beyond that, as we understand these new levels of the complexity of life, we also bring clarity to the question of how evolution equipped living organisms to cope with unpredictable environments. With greater knowledge of the structure of life, we can begin to imagine the wonders of medical and other discoveries that could follow.
Interested in exploring related topics?
Discover recommended TQR articles like,
- What If We Could Cure Cancer by Telling Cancer Cells to Get Better?
- Amazing Evolutionary Leaps: Observing the Rapid Evolution of Single Cell Organisms into Complex Lifeforms
- Life Is Defined by Biology, Not Physics