Among the many implications and predictions of Einstein’s general theory of relativity, gravitational waves have long been one of the most challenging to prove.
According to general relativity, space and time together resemble a fabric that is curved by the mass of heavy objects. In this context, gravitational waves would represent ripples in this fabric of the universe caused by massive objects with extreme acceleration.
To observe these ripples, we must detect events like the collision of black holes or neutron stars, that occur extremely far from Earth. This is an extraordinary challenge because such effects are diluted by the distance between the event and our detectors, making them incredibly difficult to measure. Yet we have managed to do so only a century after Einstein’s prediction.
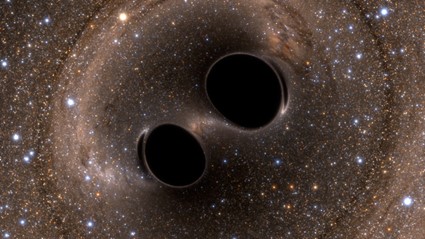
The collision of two black-holes.
Beyond their existence, the predicted effects of gravitational waves on spacetime still await experimental confirmation.
General relativity predicts that gravitational waves should permanently distort the fabric of spacetime, leaving a record of their action. Since the waves were first detected, researchers have tried to measure this memory effect. And although we have not yet reached the goal, the data suggest that we are rapidly approaching the end of this journey.
The Laser Interferometer Gravitational-Wave Observatory (LIGO) is a giant detector used to study gravitational waves and their effects on spacetime. The structure consists of two 4-kilometer-long arms arranged in an L-shape. Laser beams bounce back and forth along the arms, with advanced stabilizers, vacuum systems, and thousands of sensors enabling LIGO to detect minuscule changes in the length of the arms.
The peculiar shape of the detectors is also key to their ability to detect gravitational waves. Imagining the intersection of the arms as the center of a circle with an initial radius equal to their length, gravitational waves distort the circle as they pass through the detector. These distortions change the relative size of the arms, causing the difference between them to oscillate and reveal the change in the circumference.
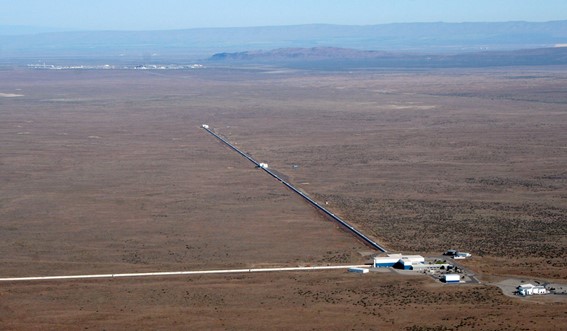
The Laser Interferometer Gravitational-Wave Observatory (LIGO).
The property responsible for the memory effect is the gravitational potential.
In classical Newtonian mechanics, gravitational potential represents the amount of energy an object would gain if it fell from a certain height. In general relativity, however, this potential goes beyond the energy at a location and determines the shape of spacetime itself. The memory effect is the change in the gravitational potential of spacetime caused by the passage of a gravitational wave.
The ways in which a gravitational wave can distort spacetime are infinite but, surprisingly, in some ways equivalent. Even if the waves upset the configuration of the fabric of spacetime as they pass, the elements of the fabric remain the same. And regardless of the configuration, these elements will always rest in the state with the lowest energy.
This equivalence between the various possible configurations of spacetime points to hidden symmetries in its structure that have increasingly interested physicists for at least 60 years.
The symmetries of the universe, derived from Einstein’s special theory of relativity and Emmy Noether’s Theorem, are called translational, rotational, and boost symmetries. These symmetries are expected in a flat and featureless spacetime. These properties mean that in the absence of mass or energy, everything looks the same regardless of where you are, which direction you are facing, or at what speed you are moving.
“Albert Einstein’s 1905 theory of special relativity is one of the most important papers ever published in the field of physics. Special relativity is an explanation of how speed affects mass, time and space. The theory includes a way for the speed of light to define the relationship between energy and matter – small amounts of mass (m) can be interchangeable with enormous amounts of energy (E), as defined by the classic equation E = mc^2. […] As an object approaches the speed of light, the object’s mass becomes infinite and so does the energy required to move it. That means it is impossible for any matter to go faster than light travels.” Space.com.
“Noether’s theorem or Noether’s first theorem states that every differentiable symmetry of the action of a physical system with conservative forces has a corresponding conservation law. The theorem was proven by mathematician Emmy Noether in 1915 and published in 1918, after a special case was proven by E. Cosserat and F. Cosserat in 1909. […] This theorem only applies to continuous and smooth symmetries over physical space.” Wikipedia.
To better understand general relativity, physicists conceived and studied the symmetries in a hypothetical region infinitely distant from all mass and energy in the universe, where the effects of gravity would be negligible.
This region is referred to as “asymptotically flat spacetime.” Unexpectedly, their models revealed an infinite number of symmetries, indicating that individual sections of spacetime could be stretched, compressed, and sheared without any change in behavior in this infinitely distant region.
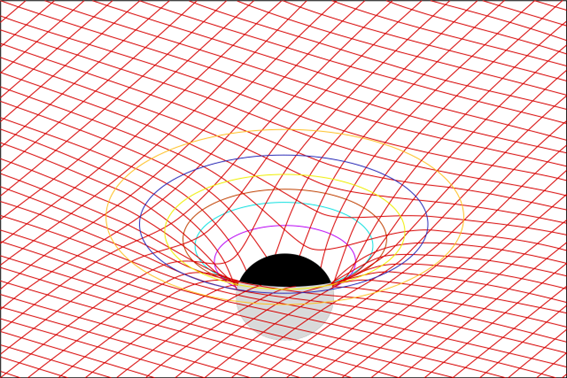
The mass of the object determines how much it bends the fabric of spacetime.
Dr. Abhay Ashtekar, a professor at Pennsylvania State University, was the first to recognize that the memory effect was a manifestation of these symmetries. This realization connected the abstract symmetries of a hypothetical region of the universe to real effects observed in our world.
Dr. Laura Donnay, a physicist at the Vienna University of Technology, declared, “To me, that’s the exciting thing about measuring the memory effect- it’s just proving these symmetries are really physical.”
Another remarkable implication of our growing understanding of the behavior of spacetime through gravitational waves is that it may resolve a long-standing paradox in physics – the black hole information paradox.
In the 1970s, the theoretical physicist Dr. Stephen Hawking discovered that black holes emit radiation in a way that is independent of their initial state. However, this implied that different physical states could evolve into the same state, meaning that information could disappear in a black hole. This contradicted a central principle of both classical and quantum physics, namely that information cannot disappear.
In 2016, Dr. Andrew Strominger, a professor at Harvard University, in collaboration with Dr. Malcolm Perry and Dr. Hawking, discovered that the same type of symmetries of asymptotically flat spacetime are present in the horizon of black holes, where we can therefore also expect the presence of the memory effect. This discovery was a response to the paradox that the information of particles that fall into black holes is permanently encoded in the fabric of spacetime.
However, given the unpaired experimental challenges of directly observing gravitational waves and their effects, the memory effect has not yet been observed.
While the state-of-the-art LIGO can detect changes as small as one-thousandth the width of a proton caused by the passage of gravitational waves, the predicted memory effect is 20 times smaller than that. This level of sensitivity is still far from what current technology can achieve.
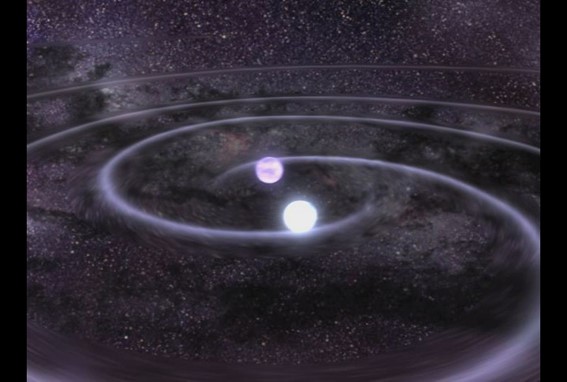
Illustration of the gravitational waves caused by the merging of two neutron stars.
In addition, scientists must work around several sources of error in detecting the effects of gravitational waves. Low-frequency seismic noise changes the positions of the mirrors in the detector, mimicking the memory effect. Meanwhile, Earth’s gravity returns the mirrors to their original location because although the distortions in spacetime are permanent, the changes in the mirrors are not. Thus, scientists must filter out the noise in the data and detect the effect of the waves before Earth’s gravity resets the detector.
But despite current technological limitations, we may not have to wait too long to detect the memory effect of gravitational waves.
Researchers like Dr. Patricia Schmidt, a professor at the University of Birmingham, and Dr. Paul Lasky, a professor at Monash University, suggest that we could stack data from multiple waves and detect their summed effects. They estimate that we would need more than 1,000 gravitational waves to confirm that the expected memory is present, which may be only a few years away, given the ongoing research efforts in this area worldwide.
Beyond a glimpse of the effects of the elusive gravitational waves, this area of research explores how matter, energy, and spacetime are interconnected. The memory effect caused by the passage of waves through the fabric of spacetime hints at the hidden symmetries of the fibers that make up our universe. It may prove to be the ultimate connection that seams the universe, all the way from Earth to hypothetical regions that are infinitely distant from here.