
“Levitation of a magnet on top of a superconductor”. Image: Julien Bobroff
By James Myers and Mariana Meneses
Last year, a group of scientists claimed to have created a material, named LK-99, that was a room-temperature, ambient-pressure superconductor. The claim was met with scepticism by the scientific community and subsequent investigations disproved it. However, there is a reason for all this hype around superconductivity, and progress is, indeed, being made.
A room-temperature ambient-pressure superconductor is a theoretical material that can conduct electricity with zero resistance at everyday temperatures and normal atmospheric pressure.
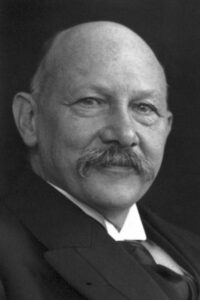
Heike Kamerlingh Onnes discovered superconductivity in mercury. Image: Nobelprize.org
Current superconductors require either extremely high pressure or cooling to very low temperatures, often close to absolute zero, because only at these conditions does the electrical resistance of the materials drop to zero. Cooling or high pressure are necessary to overcome the disruptive thermal energy that interferes with the pairing of electrons needed to move through a superconductor without the resistance that arises when electrons otherwise normally repel each other.
The phenomenon of superconductivity was discovered in 1911 by Heike Kamerlingh Onnes, after chilling a piece of mercury wire. Two years later, Kamerlingh Onnes was awarded the Nobel Prize for producing a liquid version of helium, the gas that powers stars, exhibiting the characteristics of a superfluid with zero viscosity. Liquid helium is currently used, for example, for cooling in MRI machines which could benefit from superconductivity.
Physicist Siddharth Shankar Saxena has recently discussed the transformative potential of superconductors in eliminating energy scarcity and revolutionizing quantum computing. By enabling electrical transmission without resistance, superconductors could vastly improve energy efficiency and transform transportation, communication, data storage, and power grids on a global scale.
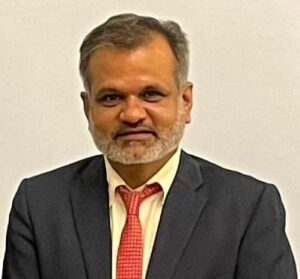
Dr. Siddharth S Saxena, Principal Research Associate at the University of Cambridge’s Cavendish Laboratory and Fellow at Jesus College, is a distinguished physicist recognized internationally for his contributions to materials science and quantum complexities in condensed matter. Image: University of Cambridge.
By significantly improving energy efficiency, superconducting technologies could reduce or eliminate reliance on fossil fuels and other non-renewable resources and make a major contribution to alleviating the climate crisis.
Superconductivity is already producing economic consequences.
For instance, the global market for superconductor wires, which conduct electricity with almost no resistance when cooled to very low temperatures, is projected to grow from $605.9 million in 2022 to an estimated $1.2 billion by 2031. These wires are crucial in applications ranging from MRI machines to smart grid technologies and energy storage systems, offering significant advantages over traditional cables in terms of efficiency and voltage capacity.
As their use expands across various industries, the market for superconducting wires is poised for substantial growth in the coming years.
Some experts caution, however, that superconductors might not be a panacea because of barriers to practical implementation that include obtaining investment capital to replace existing equipment and engineering the superconducting materials into ductile and flexible wires. They argue that if current loss in energy grids amounts to 5%, there could be more effective means to increase energy efficiency; others, however, estimate energy loss during transmission to be as high as 15%.
Superconductivity could also provide significant benefits in areas such as medical technologies.
Operating a magnetic resonance imaging (MRI) machine, which generate powerful magnetic fields to produce three-dimensional images of the body’s internal structures, require a costly 500 gallons of helium for cooling which could be eliminated by superconductors without sacrificing high-resolution image quality.
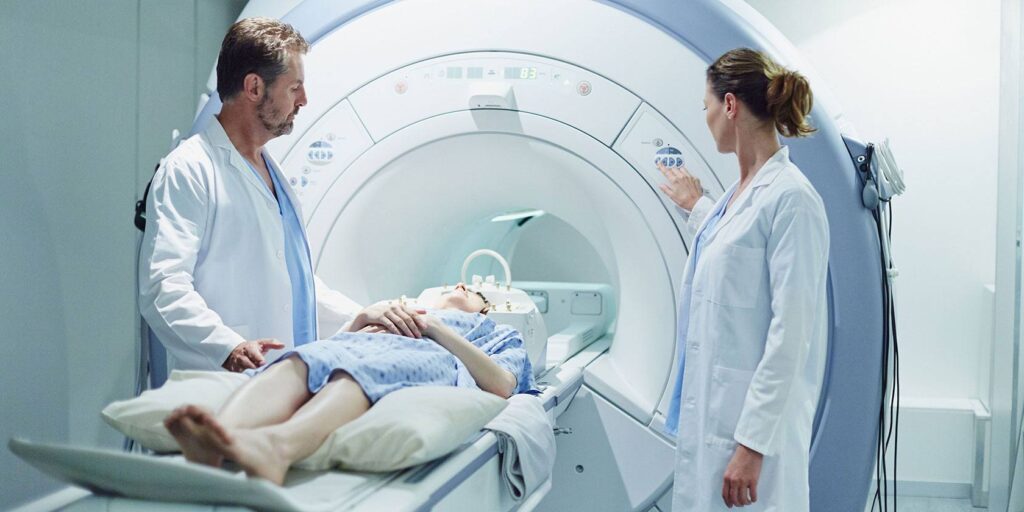
A patient is prepared for an MRI scan of the brain. A painless, non-invasive procedure, MRI (Magnetic Resonance Imaging) technology uses strong magnetic fields that force alignment of protons in the tissues to produce a 3D map of the brain. Image: First Look MRI
Superconductivity holds significant promise for quantum computing.
In quantum computing, superconductors could eliminate resistance and address the challenging issue of error correction, paving the way for more reliable and powerful quantum computers with unprecedented computational capabilities.
In quantum computing, error correction is crucial due to the delicate nature of quantum states. Quantum bits, or qubits, have to maintain two energy states simultaneously but are prone to errors from environmental disturbances such as heat from electrical resistance which can collapse the qubits into the lowest energy state. These errors can lead to decoherence, a process where the quantum circuits lose their connections due to interactions with the surrounding environment, resulting in the deterioration of unique properties of superposition and entanglement that make the quantum computer so powerful.
This decoherence disrupts the complex probabilities that define qubit states, necessitating robust quantum error correction methods to preserve quantum information for accurate computation and the development of functional quantum computers.
Interviewed by Janna Levin for Quanta Magazine’s The Joy of Why Podcast, Dr. Saxena highlighted ongoing research aimed at discovering new superconducting materials that could operate at higher temperatures, making their applications more feasible and widespread.
The Cavendish Laboratory, where Dr. Saxena is Principal Research Associate, is conducting advanced studies on superconductors, magnets, graphite, and renewable energy. Using experimental approaches involving high-pressure and low-temperature conditions, the lab seeks to identify new materials and understand the underlying physics that could enable significant advancements in energy transmission and medical technologies.
Dr. Saxena also discussed the ongoing interdisciplinary efforts combining physics, materials science, and engineering to overcome current limitations and bring the theoretical potential of superconductors into practical, everyday use, ultimately aiming to address global energy challenges and push the boundaries of computational technology.
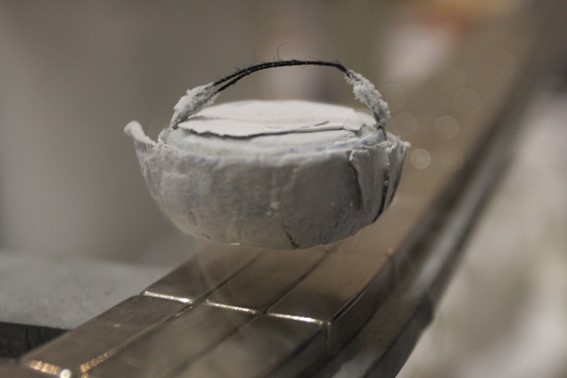
Superconductor levitating above a magnet. The electrical field surrounding the superconductor excludes the magnetic field from the magnet in a process known as the Meissner effect. Image: Henry Mühlpfordt, on Wikipedia.
Research on superconductivity in quantum computing is making progress.
Topological quantum computing stands to benefit from advances in superconductivity. Our February article, The Geometry of Information: Is Topological Quantum Computing the Future? described developments in this type of quantum computing, in which information is encoded globally in the system and them tracked through the shape, or topology, of the signals as they move through space and time. A class of superconductors, called topological superconductors, can be used to generate qubits that are not prone to decoherence from environmental disturbances, which could make topological quantum computing much more reliable.
Discovered five years ago, a material called uranium ditelluride (UTe2) is a potential superconductor that holds promise for encoding quantum information in the rotation of electron pairs, called Cooper pairs. Scientists led by Dr. Séamus Davis of University College Cork, in Ireland, have succeeded in measuring the unique rotational properties and varying densities of Cooper pairs of electrons in UTe2, indicating the possibility of recording quantum information in the resulting topological shapes.
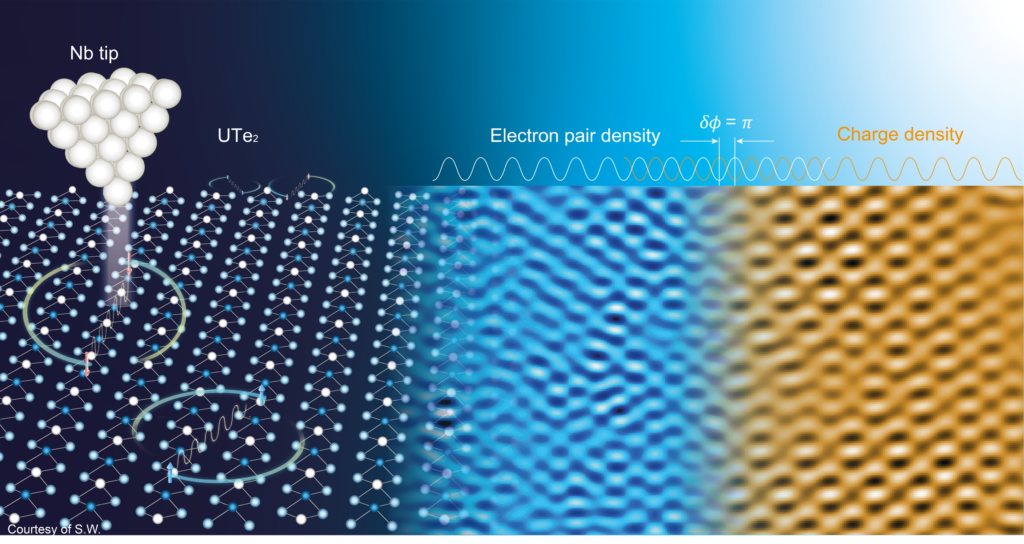
Detection of an electron “Pair Density Wave: state in uranium ditelluride, a superconducting compound, could exhibit “many unprecedented electronic properties including fractionalized electronic states relevant to quantum information processing.” Image: The Davis Group
As University College Cork PhD researcher Joe Carroll explained to Advanced Science News, “In such materials, there is no limit on the lifetime of the qubit during computation, opening up many new ways for more stable and useful quantum computers.”
In another development relating to superconducting qubits, an international group of researchers has proposed the use of a superconducting copper-based material called a cuprate in quantum computing circuits. To operate correctly, superconducting qubits require a gap in the circuit called a Josephson junction, where the “Josephson effect” occurs in which Cooper pairs of electrons can tunnel through an insulating barrier without any voltage applied.
The result is a superconducting current that that flows without resistance while still subject to control by the Josephson junction, although the time required for the electrons to pass through the gap increases the probability of the qubits decohering. The proposal is for the use of atom-thick flakes of the superconducting cuprate at a 45-degree angle in the Josephson junction to overcome the disturbance the qubits would otherwise experience during the transit time in the Josephson junction.
Interesting progress is also being made among physicists at the RIKEN Center for Emergent Matter Science, who have created and studied quantum-mechanical states resembling molecules, known as Andreev molecules, in superconducting circuits. These states could have significant applications in future quantum computers, given that “coupling individual atoms via tunneling fundamentally changes the state of matter” and the process of Andreev reflection in the scattering of particles allows for the connection of a superconductor to a regular material.
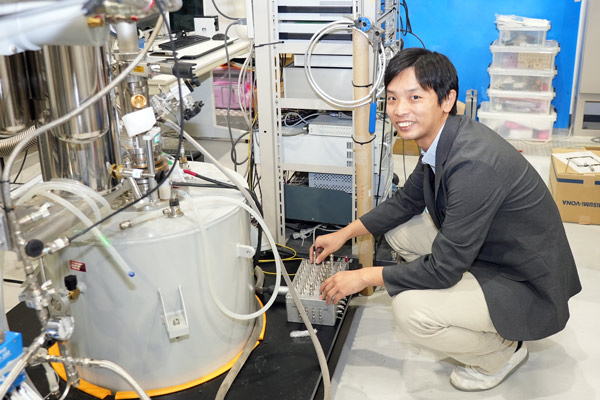
“The devices we make help provide information on superconducting phenomena peculiar to its structure, but sometimes the phenomena may also reflect more universal properties in physics. I like to spend time considering unusual device structures and their physical properties. It would be an added pleasure to reveal some important fundamental physics related to these structures.” – Sadashige Matsuo. Image: Sadashige Matsuo/RIKEN.
The research team, led by Sadashige Matsuo, created a structure with two Josephson junctions sharing a superconducting aluminum electrode.
They observed that the Andreev bound states in the junctions linked through the shared electrode, enabling one junction to control the other and giving rise to novel superconducting phenomena. By using tunneling spectroscopy, they measured the energy levels of these Andreev molecules under various conditions. This work marks the first demonstration of controllable Andreev molecules in coupled Josephson junctions, providing foundational knowledge for future developments in superconducting circuits and quantum information technologies.
The advent of room-temperature and ambient-pressure superconductors would revolutionize our daily lives by significantly reducing energy consumption and costs, as electrical grids could transmit power without any losses, leading to lower energy bills. Public transportation could see a leap forward with the introduction of magnetic levitation trains that are faster and more energy-efficient due to the elimination of friction.
In healthcare, MRI technology would become cheaper and more widely available, making advanced medical imaging accessible to a broader population. Consumer electronics would benefit from quicker charging times and longer battery life, as superconductors would minimize energy waste during power transmission within devices. These advancements would not only enhance convenience but also contribute to a greener and more sustainable future.
While claims made to date about breakthroughs in room temperature ambient-pressure superconductors have proven premature, when success is finally achieved it will be truly worthy of the headlines.
Craving more information? Check out these recommended TQR articles:
- Race for Post-Quantum Cryptography: Will Proposed Encryption Standards Secure the World’s Data?
- What Will a Recent Quantum Leap in Time Crystal Technology Reveal About the Elusive Nature of Time?
- Adaptive Intelligence: The Power of Genetic Mutations and Neural Networks
- Citizen Action Combines With Innovative New Methods to Combat Cybersecurity Threats
- The Incredible Power of Shape: Fractals Connect Quantum Computers to the Human Body and the Cosmos