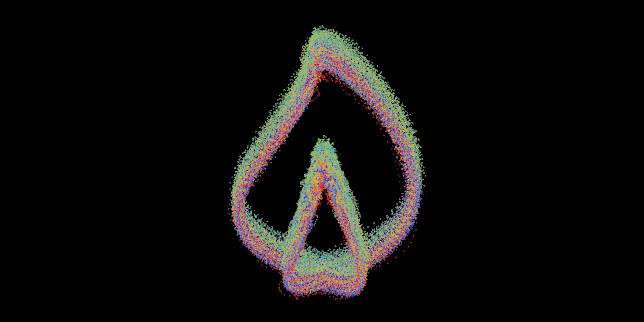
“What looks like a flame is the measurement of the new time crystal: Each point corresponds to an experimental value, resulting in different views of the periodic dynamics of the nuclear spin polarization of the time crystal.” Image: Dortmund University
By Mariana Meneses
Dr. Federico Carollo, from the University of Tübingen, in Germany, and co-authors, recently investigated the quantum thermodynamics of experimental new structures called boundary time crystals, in the journal Quantum Science and Technology.
The research probes questions about the nature of energy exchanges in thermodynamics. How do time crystals, which continually switch between quantum states without consuming energy, behave in real-world conditions, particularly when they interact with their surroundings at different temperatures? What technologies will emerge as the science of time crystals evolves?
By analyzing thermodynamic factors like heat flow and energy exchange, the research advances understanding of both the costs and potential applications of these intriguing time crystal structures for promising technologies like quantum sensing. Our December 2023 article Quantum Sensing’s Revolutionary Potential explored possible technological applications for cancer treatment, navigation, and precision measurement.
“Thermodynamics is the study of the relations between heat, work, temperature, and energy. The laws of thermodynamics describe how the energy in a system change and whether the system can perform useful work on its surroundings.” (Britannica)
The thermodynamic theory of computation is a framework that explores the energy costs involved in running computational processes. It combines principles from physics and computer science to understand and calculate the minimum and maximum energy required for different types of computations, including those influenced by randomness. This theory aims to make computing more energy-efficient by predicting the energy required for various tasks and identifying ways to reduce energy use, which is increasingly important since data-intensive computing consumes a significant portion of global fenergy production.
Understanding heat transfer is crucial for many purposes, and research is providing new insights.
For instance, physicists from the Institute of Nuclear Physics in Cracow have proposed a new wave-based theory of heat transport. It connects biological tissues to the Doppler effect, which measures the change in frequency of a sound wave as the observer moves relative to the wave’s source, and to the telegraph equations, which predict electrical voltage and current distribution in a straight line.
The study suggests that heat transport in such systems may exhibit behaviors akin to sound waves, challenging diffusion-based models predicting that waves follow a path from higher to lower concentration. This insight could potentially advance medical and cosmetic technologies by improving heat management during procedures like laser surgery and minimizing thermal effects on tissues.
Thermodynamics plays a major role in quantum computing.
As we noted in our June feature What Will a Recent Quantum Leap in Time Crystal Technology Reveal About the Elusive Nature of Time?, a significant challenge to the development of fully functioning quantum computers is the loss of connections between quantum information bits, called qubits, due to the interference of thermal equilibrium with the surrounding environment. These disconnections are called decoherence, which produces errors in the transmission of signals between qubits.
Recent advancements in time crystal technology, particularly the creation of a time crystal that can last for 40 minutes without external stimulation by scientists at TU Dortmund University, offer groundbreaking potential for technological development. Time crystals, which break the principle of time translation symmetry, could lead to extremely accurate measurements of quantum processes without disrupting them. This helps to overcome a significant challenge for measurements in quantum mechanics and computing as reported in our May feature, The Observer Effect: Why Do Our Measurements Change Quantum Outcomes?.
The breakthrough could revolutionize fields such as quantum computing and the understanding of fundamental phase transitions in the universe.
Time-crystals are special quantum phases that exhibit repetitive patterns in time even when they are not in equilibrium, which could potentially advance technologies like quantum sensing by offering stable and predictable behaviours at varying temperatures.
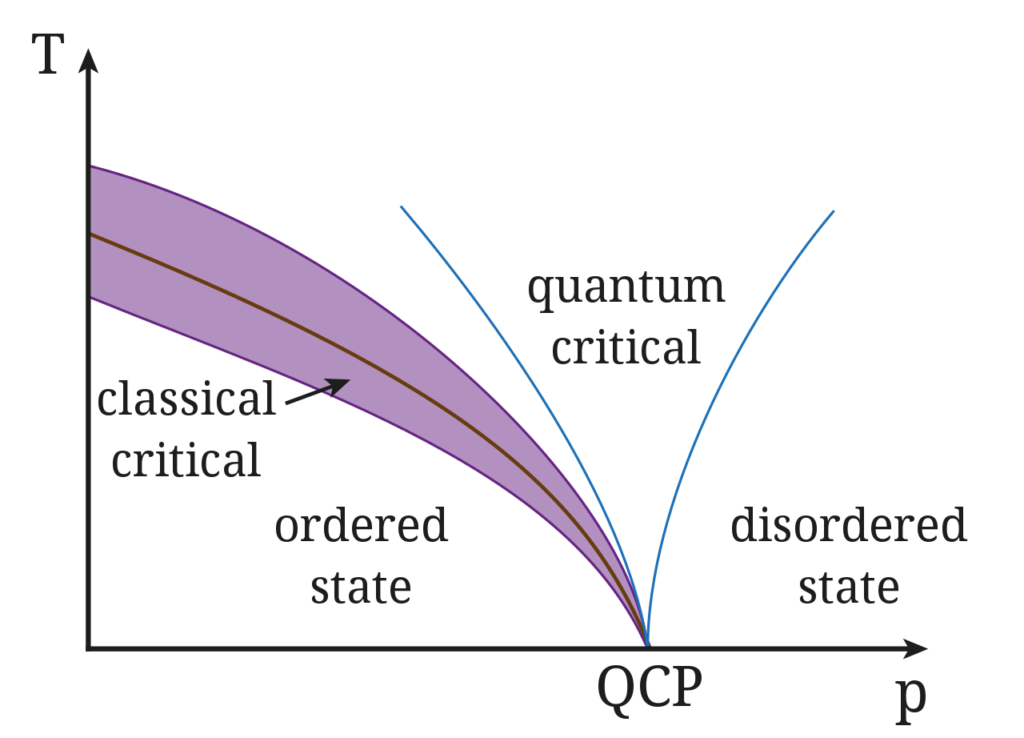
“In condensed matter physics, a time crystal is a quantum system of particles whose lowest-energy state is one in which the particles are in repetitive motion. The system cannot lose energy to the environment and come to rest because it is already in its quantum ground state.” ( Wikipedia ) Image: AG Caesar, original by DG85
The relation of time crystals to thermodynamics lies in the ability of the new experimental structures to maintain non-equilibrium states while exhibiting stable, repetitive patterns over time.
This unique property challenges traditional thermodynamic principles, particularly those related to equilibrium and entropy, which is the measure of a system’s disorder and probable states. These non-equilibrium states could significantly advance technologies like quantum computing and sensing by providing more stable and predictable behaviors at varying temperatures, thereby addressing issues such as decoherence and improving the overall reliability and efficiency of quantum systems.
Twesh Upadhyaya, a PhD candidate at the University of Maryland, explains the relationship of quantum superposition, which is the phenomenon that quanta simultaneously exhibit the same behavior when they are connected (or “entangled”), and the second law of thermodynamics which says that energy moves in one direction over time (“time’s arrow”), from hot (low entropy) to cold (high entropy).
Twesh observes that, “When two quantum systems, say, a hot and a cold atom, start off in a state of superposition, when they interact, their entropies can actually decrease. The surprising result is due entirely to quantum mechanics. To connect this back to the second law, it suggests that quantum superposition can act as a source of work to simulate reversing time’s arrow. Practically, this would have implications for future quantum devices running more efficiently, because reducing entropy is kind of like reducing friction.”
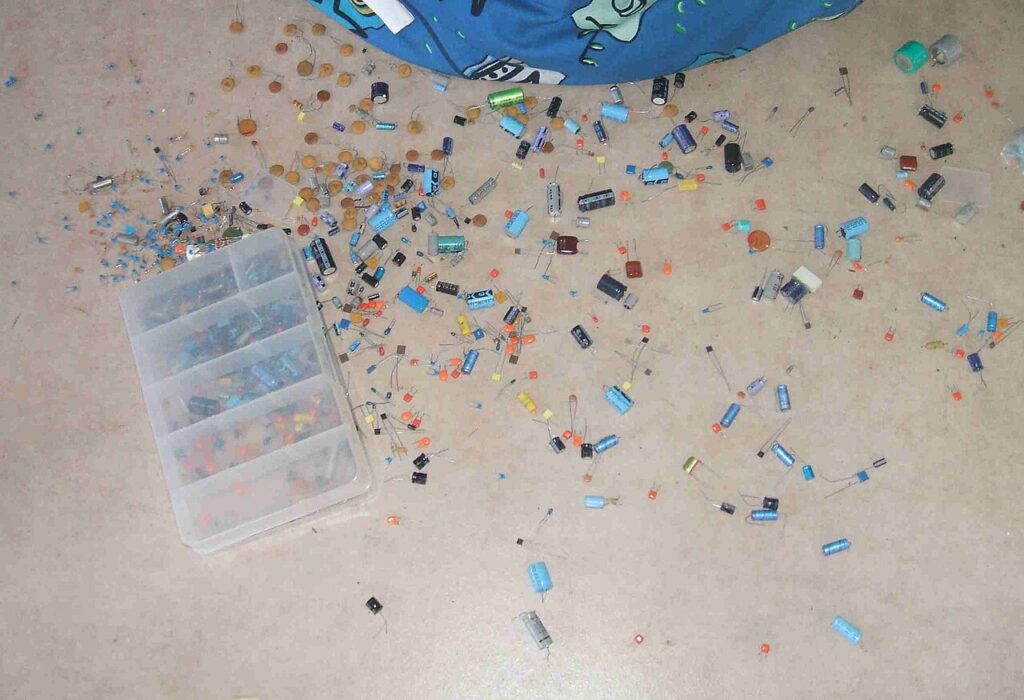
“Spontaneous increase in disorder of a carefully arranged system. An irreversible process, except with the input of energy.” The objects on the floor in this image are in a higher state of entry than when they were in their container. Credit: Alex Dinovitser
In quantum systems, the uncertainty in measurements of certain properties is linked to the amount of entropy (or probabilities) the system produces.
Increased accuracy in measurements of probabilities will improve predictions of the system’s behavior.
Recently, researchers from the RIKEN Center for Quantum Computing and the University of Amsterdam have discovered a way to make the effects of some quantum entanglements, or connections, reversible, a significant breakthrough in quantum physics. They showed that entanglement can be manipulated in a way similar to how work and heat are exchanged in classical thermodynamics, provided that success is only probable and not guaranteed. They note that, “Although such transformations have some chance of failure,” it is guaranteed that the success rate will be greater than zero.
This finding expands our understanding of entanglement and potential applications in quantum computing and communication, marking a major step forward in the field.
Thermodynamics and probability are closely related.
For instance, in a chapter of his new book, Abiogenesis: The Physical Basis for Living Systems, Laurel O. Sillerud, from the Department of Neurology at the University of New Mexico, investigates how living organisms harness energy to control the likelihood of biochemical reactions. The mechanism emphasizes the role of entropy and energy in maintaining and driving life processes such as reactions triggered by enzymes, which are proteins are essential for metabolism. In simpler terms, for example, enzymes help speed up reactions by managing the probability of molecules connecting in the right way.
Furthermore, entropy and probability are closely connected in the context of information theory and statistical mechanics.
Quantifying the amount of disorder or uncertainty in a system, entropy is directly related to the probability distribution of states in the system. Higher entropy corresponds to higher disorder or unpredictability, where many different states are equally likely and produce a high probability distribution spread, while lower entropy indicates more order or predictability, with fewer states and a lower spread in the probability distribution.
In statistical mechanics, entropy can be mathematically defined using the Boltzmann formula, which considers the number of specific configurations (microstates) given the temperature, pressure, volume, and density (the microstate) of a system. The result fundamentally relates to the probabilities of the microstates occurring. Thus, entropy and probability are intricately linked through their shared role in describing the state and behavior of physical systems.
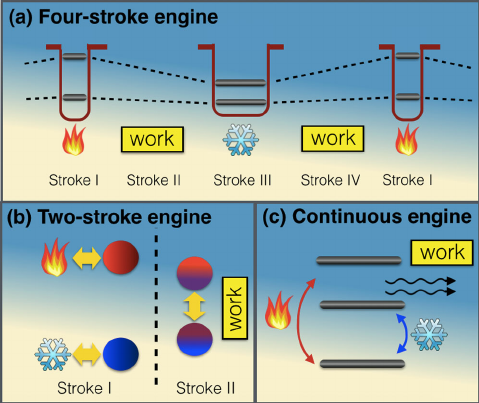
Physicists have shown that the three main types of engines (four-stroke, two-stroke, and continuous) are thermodynamically equivalent in a certain quantum regime, but not at the classical level. Credit: Uzdin, et al (2015)
The concept of entropy, as a measure of disorder or probability, is crucial in understanding how biological systems organize and maintain themselves despite the inherent randomness at the molecular level.
According to a study published by Jonathan Asher Pachter and colleagues (2024) in Nature Reviews Physics, statistical physics explores how large-scale properties of systems relate to the behaviors of their microscopic components. Traditionally focused on equilibrium states with maximum entropy, recent studies have expanded understanding of varying rates of change in systems by using tools like the Jarzynski equality and stochastic thermodynamics, which accounts for randomness. These advancements go beyond simple equilibrium conditions to infer behaviors based on dynamic paths rather than static states, to infer the trajectory, or evolution, of the states over time.
“The Jarzynski equality is an equation in statistical mechanics that relates free energy differences between two states and the irreversible work along an ensemble of trajectories joining the same states. (…) Fundamentally, the Jarzynski equality points to the fact that the fluctuations in the work satisfy certain constraints separately from the average value of the work that occurs in some process.” (Wikipedia)
Recent advancements in quantum thermodynamics and statistical mechanics reveal the profound interplay between energy, entropy, and probability, offering insights that challenge traditional equilibrium-based models. Research into time crystals and quantum entanglement transformations highlights the potential for groundbreaking applications in quantum computing and sensing by maintaining non-equilibrium states and reducing decoherence.
These discoveries underscore the significance of understanding heat transfer and energy efficiency, crucial for future technological innovations in fields ranging from medical procedures to computational processes.
Craving more information? Check out these recommended TQR articles:
- Race for Post-Quantum Cryptography: Will Proposed Encryption Standards Secure the World’s Data?
- What Will a Recent Quantum Leap in Time Crystal Technology Reveal About the Elusive Nature of Time?
- Adaptive Intelligence: The Power of Genetic Mutations and Neural Networks
- Citizen Action Combines With Innovative New Methods to Combat Cybersecurity Threats
- The Incredible Power of Shape: Fractals Connect Quantum Computers to the Human Body and the Cosmos